
한국형수치예보모델 가상관측 영향평가체계(KIM-OSSE)를 활용한 정지궤도 초분광적외탐측기(GeoHIS) 예측 영향평가
Ⓒ 2025 Korean Meteorological Society
Abstract
The Korea Meteorological Administration (KMA) will operate a hyperspectral infrared sounder based on a geostationary satellite in 2037. The next-generation Geostationary Hyperspectral Infrared Sounder (GeoHIS) observes vertical temperature and humidity with high temporal and spatial resolution. Therefore, it can be used not only directly in nowcasting but also as input data for numerical prediction model, which is expected to improve weather forecast. We analyzed the forecast impact of the next-generation GeoHIS, which has high density in the Asia region, using the Korean Integrated Model-Observing System Simulation Experiment (KIM-OSSE). As a result of the forecast impact, improvements were confirmed in the mid to upper-level geopotential height (500~250 hPa) and lower-level temperature (850 hPa) of the troposphere in the globe and the Northern Hemisphere. In particular, we confirmed a significant improvement in the mid to upper-level geopotential height in the Asia region. These results will be used as support material for advancing of satellite data assimilation technology and for the establishment of next-generation satellite launch plans.
Keywords:
Korean Integrated Model-Observing System Simulation Experiment (KIM-OSSE), Geostationary Hyperspectral Infrared Sounder (GeoHIS), Temperature and humidity sounding, Forecast impact1. 서 론
대기 연직 관측은 고도별로 기온, 습도, 그리고 바람을 관측할 수 있어 위험기상을 진단하고, 예측하는데 중요하게 쓰이는 자료 중 하나이다. 직접적인 대기 연직 관측은 라디오존데(radiosonde)를 이용하여 수행되어 왔다. 라디오존데 관측은 고정된 지점에서 하루에 2~4회(0000~1800 UTC), 주로 육상에서 관측된다. 반면, 기상위성 관측은 광범위한 영역에서 3차원 관측이 가능하기 때문에 해양을 포함한 기상감시 공백지역에 대해 조밀한 관측이 가능한 장점이 있다(Cho et al., 2014; 2017). 특히, 기상위성으로부터 산출된 고도별 온도, 습도, 그리고 바람은 대기 불안정도(instability), 연직시어(wind shear), 총가강수량(total precipitable water, TPW) 등 강수 구름의 이동 및 발달과 관련된 기상변수를 산출할 수 있어 실황예보(nowcasting)에 유용하게 사용될 수 있을 뿐만 아니라 수치예보모델 입력자료로 활용되어 예측정확도 향상을 기대할 수 있다(Yang et al., 2017; Menzel et al., 2018; Jones et al., 2020; Holmlund et al., 2021; Li et al., 2021).
2000년대부터 고품질의 AIRS (Atmospheric Infrared Sounder; Chahine et al., 2006), IASI (Infrared Atmospheric Sounder Interferometer; Hilton et al., 2009), 그리고 CrIS (Cross-track Infrared Sounder; Strow et al., 2013) 초분광적외탐측기(Hyperspectral Infrared Sounder, HIS) 관측이 수행되기 시작하였다. 공간해상도는 12~14 km, 관측폭과 채널수가 1,650~2,200 km와 약 1,305~8,461개로 높은 연직 해상도의 고품질 자료가 제공되면서 유럽중기예보센터(European Centre for Medium-Range Weather Forecasts, ECMWF), 영국 기상청(Met Office) 등 현업 수치예보모델 예측정확도 향상에 크게 기여하였다(Joo et al., 2013; Eresmaa et al., 2017; Noh et al., 2021). 이러한 3차원 위성관측은 주로 극궤도 위성(polar orbit satellite)에서 수행되어 궤도상 동일한 지역에서 일 2~3회 정도만 관측이 가능한 단점이 있다. 반면 정지궤도 위성은 적도상공에 위치하면서 동일한 지역에 대해 높은 시공간 분해능(예: 30분 간격, 4 km)으로 연속적인 관측이 가능하다. 최근 3차원 온습도 관측에 있어 극궤도 위성의 단점을 보완하기 위해 정지궤도 위성(geostationary satellite) 기반의 마이크로파탐측기(microwave sounder)와 HIS 연구들이 진행되고 있다(Iturbide-Sanchez et al., 2022; Posselt et al., 2022).
세계기상기구(World Meteorological Organization, WMO)는 2 0 40년까지 최소 5기 정지궤도 초분광적외 탐측기(Geostationary HIS, GeoHIS) 운영을 목표로 하는 “Global ring”을 비전으로 제시하였다(WMO, 2019; Li et al., 2022). 중국은 GIIRS (Geostationary Interferometric Infrared Sounder)와 GIIRS2 센서를 각각 2 0 16년과 2021년에 FY-4A (FengYun-4A)와 FY-4B에 탑재하여 운영 중에 있고, 유럽은 2025년에 IRS (InfraRed Sounder)를 탑재한 MTG-S1 (Meteosat Third Generation-Sounder1)를 발사하여 2 0 26년에 운영할 계획에 있다. 또한 일본과 미국은 GHMS (Geostationary HiMawari Sounder)를 탑재한 Himawari-10과 GXS (GeoXO Sounder)를 탑재한 GeoXO (Geostationary Extended Observations)를 각각 2 0 29년과 2 0 36년에 운영할 계획에 있다(Table 1; Yang et al., 2017; Adkins et al., 2021; Bessho et al., 2021; Holmlund et al., 2021; Iturbide-Sanchez et al., 2022).
기상청(Korea Meteorological Administration, KMA) 국가기상위성센터(National Meteorological Satellite Center, NMSC)는 2 0 29년부터 GeoHIS를 개발하고, 2036년에 발사 및 시험을 거쳐 2037년에 현업 운영을 계획하고 있다(NMSC, 2023). GeoHIS는 높은 시공간 분해능으로 대기운동벡터, 대기 연직온도, 수증기량 등 역학적(dynamic) 그리고 열역학적(thermodynamic) 대기 변수를 관측할 수 있다. 위험기상을 탐지하는데 있어 기상레이더의 경우, 강수입자가 생성되어야 관측이 가능하고, 기상위성 적외 및 가시영상기(imager)는 구름이 생성되어야 관측이 가능하다. 그러나 GeoHIS는 위험기상이 발생하기 전 맑은 하늘에서 연직 불안 정도 등 위험기상이 발생할 수 있는 대기의 상태를 관측할 수 있다. 따라서 기상레이더, 기상위성 영상기 보다 위험기상을 사전에 탐지할 수 있어 예보 선행시간이 더욱 확보될 수 있을 것으로 기대된다. 이러한 정보는 고스란히 수치모델 입력자료로 활용되어 위험 기상 예측정확도 향상을 기대할 수 있을 것이다.
GeoHIS와 같이 현존하지 않는 차세대 위성관측에 대한 수치예보모델 영향평가 연구는 주로 가상관측영향평가체계(Observing System Simulation Experiment, OSSE)를 활용하여 수행되어 왔다(Arnold et al., 1986; Atlas, 1997; Hoffman and Atlas, 2016; Zeng et al., 2020; Cucurull et al., 2024). GeoHIS 수치모델 영향 평가 연구에서 Holmlund et al. (2021)은 유럽 정지궤도 초분광적외탐측기(IRS/MTG-S) OSSE 연구를 통해 바람 예보에서 매우 긍정적인 영향을 주었다고 보고하였고, 따라서 IRS처럼 높은 시공간 분해능을 지닌 GeoHIS가 수치모델에 매우 긍정적 영향이 예상된다고 강조하였다. McGrath-Spangler et al. (2022)는 미국 차세대 위성인 GXS/GeoXO에 대해 OSSE 연구를 수행한 결과, GeoHIS 자료동화가 질량 및 바람장 개선에 긍적적인 효과가 있음을 확인하였다. 특히 적도 지역에서 뚜렷하였고, 또한 24~48시간 예측에서 오차가 감소하였다고 보고하였다. 일본에서는 GeoHIS의 잠재적 영향을 분석하기 위해 재분석자료(ECMWF Reanalysis v5, ERA-5)를 활용하여 가상관측자료를 생산하고, 수치모델 영향을 분석하였다. Okamoto et al. (2020)은 남반구 고위도 지역에서 온도와 바람의 72시간 예측에 개선이 있음을 확인하였고, Fujita et al. (2023)은 바이우 전선(Baiu front)과 상층골(upper-level trough) 예측 성능 개선을 보고하였다.
기상청은 2 0 20년 4월 28일부터 독자 개발(2011~2019년)한 한국형수치예보모델(Korean Integrated Model, KIM; Hong et al., 2018)을 현업 운영 중에 있고 WMO “Global ring” 비전에 맞추어 우리나라뿐만 아니라 세계 주요 기상선진국에서 GeoHIS를 운영 및 지속적으로 계획하고 있다. 따라서 우리 나라에서 독자 개발한 한국형수치예보모델에 차세대 위성관측인 GeoHIS 활용체계를 구축하고, 수치모델 예측 영향평가를 선제적으로 수행할 필요가 있다. 본 연구에서는 한국형수치예보모델 기반 가상관측 영향평가체계(KIM-OSSE)를 활용하여 차세대 정지궤도 위성에 탑재될 초분광적외탐측기(GeoHIS)에 대한 한국형모델 예측 영향평가를 수행하였다. 2장에서는 자료 및 방법을 서술하였고, 3장에서는 GeoHIS 가상관측자료 생산 및 영향평가 결과, 그리고 4장에서는 요약 및 결론 순으로 서술하였다. 본 연구 결과는 차세대 기상위성 발사 계획 수립 시 정책지원뿐만 아니라 한국형수치예보모델 위성자료동화 기술 고도화 연구의 기초자료로 활용될 것이다.
2. 자료 및 방법
기상청 국가기상위성센터에서 2 0 36년에 발사 계획중인 차세대 정지궤도 초분광적외탐측기(GeoHIS)의 가상관측자료 생성 및 한국형수치예보모델 예측 영향평가를 수행하기 위해 차세대수치예보모델개발사업단(Korea Institute of Atmospheric Prediction System, KIAPS)에서 개발된 KIM-OSSE를 활용하였다(Han et al., 2023). KIM-OSSE는 가상관측자료를 이용한 한국형수치예보모델 순환실험체계로 차세대 관측에 대한 영향평가를 수행할 때 주로 사용된다. Figure 1은 연구 흐름도를 보여준다. KIM-OSSE 체계는 크게 Nature Run (NR) 자료를 이용한 가상관측자료 생산과 가상관측의 수치모델 영향평가를 위한 한국형수치예보모델 순환실험, 그리고 결과 검증으로 구분되어진다.
가상관측자료를 산출하기 위해 사용된 입력자료(NR)는 콜로라도 주립대학교(Colorado State University, CSU) CIRA (Cooperative Institute for Research in the Atmosphere)에서 배포된 ECO1280 (ECMWF Cubic Octahedral1280) 자료이다. ECO1280 자료는 ECMWF (IFS cylcle 43r1) 모델에서 생산되었고, 격자체계는 cubic octahedral로 수평해상도와 연직층 수는 각각 9 km와 137층이다. 배포된 자료 기간은 14개월(2015년 9월 30일~2016년 11월 30일)로 2 0 15년 10월 한달 간은 1시간 간격 자료이고, 그 외 기간은 3시간 간격으로 구성되어져 있다(Table 2; Hoffman et al., 2019). 본 연구에서는 GeoHIS를 포함한 지상, 고층기상관측, 항공기, GNSS (Global Navigation Satellite System) 적외 및 마이크로파 복사량 등 총 18종의 종관 및 위성 가상관측자료를 생산하기 위해 10월 한달 간의 1시간 간격 ECO1280 NR 자료를 이용하였다(Table 3).
차세대 정지궤도 초분광적외탐측기(GeoHIS) 가상관측자료를 생산하기 위해 적용된 관측 특성은 위도 0oN, 경도 128.2oE로 한반도 상공에서 관측을 수행하는 천리안2호(Geostationary Korea Multi-Purpose Satellite-2A, GEO-KOMPSAT-2A, GK-2A)의 관측 특성을 적용하였고, 관측 파장대는 GIIRS/FY-4A 센서 특성을 적용하였다(Fig. 2). Figure 2b는 GIIRS 센서와 AIRS, CrIS, IASI, IRS 등 초분광적외탐측기 센서들의 관측 파수(wavenumber) 범위를 보여준다. GIIRS 센서는 3차원 연직 온습도 관측을 위해 이산화탄소(CO2)와 수증기(H2O) 흡수대가 위치한 장파적외(longwave infrared) 영역인 7 0 0 ~ 1,130 cm-1 (8.85~14.29 μm)와 중적외(midwave infrared) 영역인 1,650~2,250 cm-1 (4.44~6.06 μm) 파수(파장) 범위에서 복사량을 관측한다. 복사량 관측 채널수와 복사 분해능은 약 1,650개와 0 .625 cm-1이다. GeoHIS 복사량 산출에 사용된 복사전달모델(radiative transfer model, RTM)은 RTTOV (Radiative Transfer for TIROS Operational Vertical Sounder) 버전13을 이용하였다(Hocking et al., 2021).
GeoHIS 가상관측자료의 전처리(preprocessing) 과정과 채널선정(channel selection)은 KIAPS에서 구축된 GIIRS/FY-4A 전처리 조건을 적용하였다. 주요 전처리 과정에는 크게 구름탐지, 편향보정, 그리고 솎아내기가 적용된다. 구름에 오염된 복사량 자료를 제거하기 위해 ECMWF에서 개발된 구름탐지(cloud detection) 기법이 적용되었다(McNally and Watts, 2003; Eresmaa, 2014). 자료의 공간적 불연속성, 대기밀도 영향 등을 고려하여 편향보정(bias correction)이 적용되었고, 솎아내기(thinning) 간격은 3.0o로 적용되었다. 채널선정은 수치예보모델에서 연직 온도 관측이 중요하게 사용되기 때문에, 본 연구에서는 이산화탄소(CO2), 수증기(H2O), 그리고 오존(O3) 흡수대가 위치한 700~1,130 cm-1 (8.85~14.29 μm) 파수(파장) 범위에 분포하는 총 69개(ch1~629번) 채널이 선정되었다(Kim and Kang, 2022a, 2022b). GeoHIS 가상관측자료의 공간과 시간 분해능은 각각 16 km 그리고 1시간 간격으로 생산하였다(Table 4).
GeoHIS 가상관측자료를 활용하여 KIM의 예측 성능 평가를 수행하였다. 모델 해상도는 24 km (NE180)이고, 자료동화체계는 H4DEV (Hybrid 4D Ensemble Variational Data Assimilation)로 공간해상도는 5 0 km (NE090)이다(Table 5). 1시간 간격의 GeHIS 가상관측자료의 예측 영향평가를 위해 동일 간격의 NR 자료가 존재하는 10월을 연구기간으로 선정하였다. 모델수행기간은 2 0 23년 9월 30일~10월 30일이고, 검증기간은 스핀업 타임과 120시간 예측 평가를 고려하여 2023년 10월 5일~25일로 총 21일을 설정하였다. 실험은 크게 GeoHIS 활용 여부를 조건으로 CTL (17종; conventional + satellite)와 EXP (18종; CTL + GeoHIS)를 수행하였다. GeoHIS 예측 오차 개선율(improvement rate, %)을 계산하기 위해 NR 자료를 기준으로 EXP와 CTL 각각의 평균제곱근오차(root mean square error, RMSE)를 계산하였고, EXP와 CTL을 비교하여 오차 개선율(%)을 계산하였다. 계산식은 다음과 같고, 개선율(%)이 양의 값일 때 개선됨을 의미한다.
본 연구는 한반도를 중심으로 관측을 수행하는 GeoHIS의 수치모델 영향을 분석하기 위해 검증영역을 총 6개 지역으로 구분하여 개선율을 계산하였다. 검증영역은 전구, 북반구(위도: 20~90oN, 경도: 0~360oE), 적도(20oS~20oN, 0~360oE,), 남반구(20~90oS, 0~360oE,), 아시아(25~65oN, 60~145oE), 그리고 동아시아(20~55oN, 100~150oE) 지역이고, 전구, 북반구, 아시아, 그리고 동아시아 지역을 중점으로 세부 분석을 수행하였다(Fig. 3).
3. GeoHIS 가상관측자료 생산 및 한국형수치 예보모델 예측 영향평가
GeoHIS를 포함하여 종관 및 위성의 가상관측자료를 생산하기 위해 10월달 1시간 간격 ECO1280 NR 자료를 활용하였다. ECO1280 자료의 검증을 위해 Han et a l. (2023)과 Cucurull et al. (2024)는 강수, 온도, 그리고 바람에 대해 분석을 수행하였다. Han et al. (2023)은 2015년 10월 GPCP (Global Precipitation Climatology Project) 전구 월평균 강수량 자료를 활용하여 ECO1280 강수량과 비교한 결과, 유사한 패턴을 확인하였다. 또한 Cucurull et al. (2024)는 ERA-5 자료를 활용하여 검증을 수행하였다. 2016년 7월 한달 동안 동서(u) 및 남북(v) 방향 바람벡터와 온도의 동서 평균(zonal mean)을 비교하여 유사한 패턴을 확인하였다.
ECO1280 NR 자료를 활용하여 1시간 간격의 GeoHIS 가상관측자료 생산하였다. 관측 파장대와 위치는 각각 GIIRS 센서의 온도관측 영역과 천리안2A호 위성의 특성을 적용하였다. Figure 4는 초분광적외탐측기 6번 채널(703.125 cm-1)에 대한 가상관측(simulated observation), 섭동(perturbation), 그리고 참값(true)으로 섭동과 참값을 합하여 가상관측자료를 생산하게 된다. 섭동의 표준편차 값은 1.5 K으로 최소와 최댓값은 -6.85 K과 8.29 K을 보였다. 채널 6번은 대류권 상층 온도를 관측하는 채널로 섭동과 참값을 합한 가상관측의 최소, 최댓값 그리고 평균값은 각각 204.1 K, 233.4 K, 그리고 222.8 K, 표준편차는 2.9 K을 보였다.
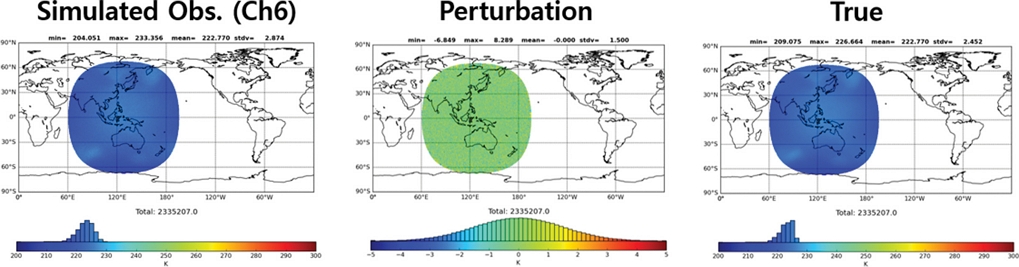
Simulated brightness temperature (Ch6, 703.125 cm-1) at GK2A position at 0000 UTC 1 October 2023 for the upper-level temperature sounding in the troposphere (Simulated observation = Perturbation + True).
GeoHIS의 관측 주기는 1시간 간격으로 동일한 지역에서 고정된 시간에 하루 2~3회 관측하는 극궤도 위성에 비해 약 8~12배 정도 많은 수치이다. 참고로 IASI를 탑재한 MetOp-B (Meteorological Operational satellite-B) 극궤도 위성의 적도통과시각(equator crossing time, ECT)은 오전과 오후 9:30이다. 관측 범위의 경우, 극궤도 위성에 탑재된 초분광적외탐측기 센서(AIRS, IASI, CrIS)는 약 1,600~2,200 km이다. 반면 정지궤도 위성은 약 12,000 km (경도 60~180oE)로 약 6배에 달한다. 따라서 차후 정지궤도 위성의 3차원 관측은 관측 주기와 범위 측면에서 극궤도 위성보다 관측 밀도가 상대적으로 높아 활용도가 높을 것으로 기대된다.
GeoHIS의 자료동화 활용 조건으로 초분광적외탐측기 관측 중 대류권 하층 관측자료는 지표면 온도(skin temperature) 및 지표 방출율(surface emissivity)의 영향을 받는 채널로 자료동화에서 제외하였다. 본 연구에서는 69개 가상관측 복사량 중 고도 1.5 km 이하(850 hPa 등압면 고도) 관측자료를 제외한 700~757.5 cm-1 파수 범위에 위치하는 42개 채널(ch1~93번)을 자료동화에 활용하였다(Table 4). 이 채널들은 이산화탄소(CO2) 흡수대에 위치하는 채널들로 대류권 연직 온도를 산출하기 위해 사용된다. 또한 경사경로(slant path)의 영향을 고려하여 고위도 지역에 대한 관측자료를 제외하였다. 본 연구에서는 위성청천각이 55o 이상(Satellite Zenith Angle, SZA ≥ 55o)인 자료는 자료 동화에서 제외하였다.
GeoHIS 가상관측자료를 활용하여 한국형수치예보모델 예측 영향평가를 수행하였다. GeoHIS 활용 유무를 조건으로 각각 CTL (17종)와 EXP (18종; CTL + GeoHIS) 실험을 수행하였다. NR 자료를 기준으로 EXP와 CTL 각각의 RMSE를 계산하였고, EXP와 CTL을 비교하여 오차 개선율(%)을 계산하였다. Figure 5는 0 00 0 UTC를 기준으로 기상요소, 등압면 고도, 그리고 영역에 따른 개선율(%)과 통계적 유의수준을 보여준다. 개선율은 양의 값(녹색)일 때, 개선됨을 의미한다. GeoHIS 고도별 영향을 분석하기 위해 850, 500, 그리고 250 hPa 등압면 고도에 대해 분석을 수행하였다.
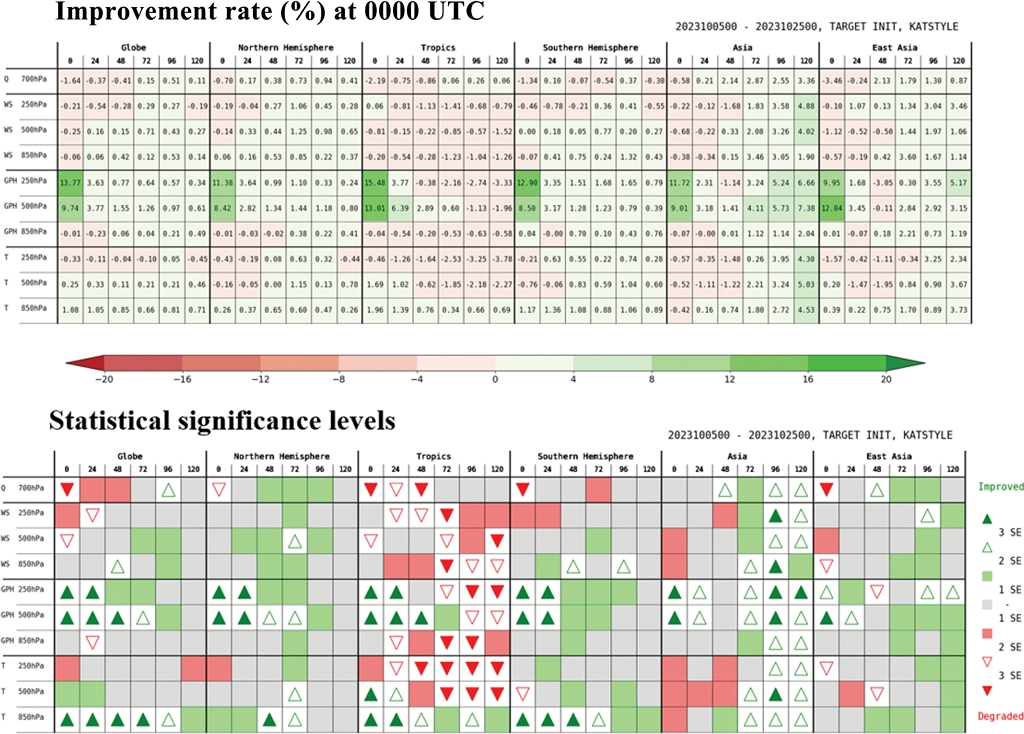
Scorecard (improvement rate, %) and statistical significance levels (1~3σ, approximately 68~99%) for atmospheric variables and regions at 0000 UTC during the period of 5~25 October 2023. T, GPH, WS, and Q denote temperature (K), geopotential height (m), wind speed (m s-1), and relative humidity (%), respectively. Positive values and green color indicate improvement.
전지구 영역에서 기상 변수에 따른 오차 개선율을 살펴보면, 지위고도, 온도, 바람, 그리고 습도 순으로 개선을 보였고, 습도는 중립에 가까운 수준을 보였다. 이것은 본 연구에서 온도 관측 영역인 700~1,130 cm-1 영역의 채널에 연구 초점을 두었기 때문에, 습도 예측 성능을 개선시키지 못한 것으로 판단된다. 지위고도는 약 1.5 km 초과 고도의 관측채널이 사용되어 중상층 지위고도 개선이 뚜렷하게 나타났고, 온도 변수에서는 850~500 hPa 고도에서 개선이 확인되었고, 250 hPa 온도에서의 개선율은 중립 수준을 보였다. 상대적으로 다른 고도와 비교하여 낮은 개선율을 보인 상층 온도는 중상층 고도의 관측채널이 활용되었음에도 불구하고 개선율이 높지 않은 것은 향후 분석이 더욱 필요한 부분이다.
주요 개선 특징을 요약하면, 전구와 북반구 지역에서 2 50 ~50 0 hPa 중상층 지위고도와 850 hPa 하층 온도에서 개선을 보였다. 또한 GeoHIS 관측 지역인 아시아 지역에서 뚜렷한 중상층 지위고도의 개선을 확인하였다. Han et al. (2019)은 중국 전구모델(China Meteorological Administration Global Forecast System, CMA-GFS)에서 GIIRS/FY-4A 장파 적외영역의 실제 관측자료를 이용하여 OSE (Observing System Experiment) 실험을 수행한 결과, 동아시아 지역 5 0 0 hPa 지위고도에서 중립 이상의 긍정적인 효과가 있음을 보고한 바 있다. 중상층 지위고도의 경우, 전구 분석장과 24~120시간 예측에서 각각 9.7~13.8%와 0 .3~3.8% 개선을 보였고, 북반구는 8.4~11.4%와 0 .9~3.6%의 rticle개선을 보였다. 특히 GeoHIS 관측 지역인 아시아는 분석장에서 9.0~13.0% 개선을 보였고, 48시간 예측(-1.1%)을 제외하고 24~120시간 예측에서 1.4~7.4% 개선을 보였다(Table 6). 95.5% 이상의 통계적 유의수준을 기준으로 5 0 0 h Pa 지위고도의 개선율을 분석한 결과, 북반구 지역에서 분석장과 예보장은 각각 8.4%, 24~72시간 예측까지 약 1.4~2.8% 개선됨을 확인하였다. 아시아 지역의 분석장은 9 .0 % 개선되었고, 예보장은 24시간 예측에서 3.2%, 72~120시간까지 4.1~7.4% 개선으로 120시간 예측까지 유의미한 개선을 보였다.

Analysis and forecast impact (improvement rate, %) at 0000 UTC based on GeoHIS data assimilation in KIM.
850 hPa 하층 온도를 분석한 결과, 전구, 북반구, 그리고 아시아 지역 24~120시간 예측에서 0.7~1.1%, 0.3~0.7%, 그리고 0.2~4.5%의 개선을 확인하였다(Table 6). 95.5% 이상의 통계적 유의수준을 기준으로 850 hPa 온도의 개선율을 분석한 결과, 북반구 지역에서 48~72시간 예측까지 약 0.6~0.7% 개선됨을 확인하였다. 아시아 지역의 예보장은 72~120 예측시간까지 1.8~4.5% 개선으로 120시간 예측까지 유의미한 개선을 보였다. 바람의 경우, 북반구와 아시아 지역 일부 예측 시간에서 개선을 확인하였고, 습도는 뚜렷한 개선을 확인하기 어려웠다. 1200 UTC를 기준으로 개선율을 확인한 결과, 0000 UTC를 기준으로 한 결과와 유사한 결과를 보였다(Fig. 6). 결과를 종합하여 볼 때, GeoHIS 가상관측자료로 전구와 북반구 지역에서 중상층 지위고도와 하층 온도의 개선을 확인하였고, 또한 GeoHIS 관측 지역인 아시아 지역에서 뚜렷한 중상층 지위고도의 개선을 보였다.
4. 요약 및 결론
기상청은 높은 시공간 분해능의 연직 온습도 관측이 가능한 초분광적외 탐측기를 탑재한 정지궤도 위성을 2 0 36년에 발사할 계획에 있다. 또한 독자 개발한 한국형수치예보모델(KIM)은 대국민 예보서비스 지원을 위해 2 0 20년 4월부터 기상청 현업모델로 운영 중에 있다. 따라서 한국형모델에 차세대 위성관측인 GeoHIS 활용 체계를 구축하고, 예측 영향평가를 선제적으로 수행할 필요가 있다. 본 연구에서는 한국형 모델 기반 가상관측 영향평가체계(KIM-OSSE)를 활용하여 차세대 GeoHIS에 대한 한국형수치예보모델예측 성능을 분석하였다. GeoHIS를 포함한 총 18종의 가상관측자료를 생산하기 위해 ECO1280 NR 자료를 이용하였다. GeoHIS 관측 특성은 한반도 상공에 위치한 천리안2A호 위치를 적용하였고, 관측 파장대는 GIIRS 센서의 특성을 적용하였다. 적외 장파영역에 위치한 총 69개의 채널에 대해 가상관측 복사량을 생산하였고, 지표면의 영향을 고려하여 1.5 km 이하 관측을 제외한 42개 채널을 자료동화에 활용하였다. 그리고 경사경로 영향을 고려하여 SZA가 55o 이상의 관측 자료는 제외하였다.
GeoHIS 포함 유무에 따라 CTL와 EXP (GeoHIS 포함)로 구분하여 순환실험을 수행하였다. 모델 수행기간은 10월 한달간 수행되었고, 검증기간은 스핀업 타임과 120시간 예측을 고려하여 10월 5~25일이다. NR 자료를 기준으로 EXP와 CTL 각각의 RMSE를 계산하였고, EXP와 CTL을 비교하여 오차 개선율(%)을 계산하였다. GeoHIS 가상관측에 대한 한국형수치예보모델 예측 영향평가를 수행한 결과, 전구와 북반구 지역에서 대류권 중상층 지위고도(500~250 hPa) 및 하층 온도(850 hPa)의 개선을 확인하였다. 특히, GeoHIS 관측지역인 아시아 지역에서 대류권 중상층 지위고도의 뚜렷한 개선을 확인하였다. 본 결과는 향후 위성자료동화 기술 고도화 및 차세대 위성발사 계획 수립 시 정책지원 자료로 활용될 것이다. 본 연구 결과는 NR 자료를 활용한 가상관측 영향평가 연구로 향후 관측오차, 관측주기, 관측영역, 관측파장대 최적화 등 실제 관측에 가까운 예측 영향평가 결과 산출을 위한 지속적인 고도화 연구가 필요하다.
Acknowledgments
This research was supported by the 「Development of Numerical Weather Prediction and Data Application Techniques (Grant No.: KMA2018-00721)」 from the Korea Meteorological Administration (KMA). We thank ECMWF for producing and CIRA/CSU for distributing the ECO1280 nature run.
References
- Adkins, J., and Coauthors, 2021: Geostationary Extended Observations (GeoXO) hyperspectral infrared sounder value assessment report. NOAA Technical Report, 103 pp.
-
Arnold, C. P., and C. H. Dey, 1986: Observing-Systems simulation experiments: Past, Present, and Future. Bull. Amer. Meteor. Soc., 67, 687-695.
[https://doi.org/10.1175/1520-0477(1986)067<0687:OSSEPP>2.0.CO;2]
-
Atlas, R., 1997: Atmospheric observations and experiments to assess their usefulness in data assimilation. J. Meteor. Soc. Japan, 75, 111-130.
[https://doi.org/10.2151/jmsj1965.75.1B_111]
-
Bessho, K., H. Owada, K. Okamoto, and T. Fujita, 2021: Himawari-8/9 follow-on satellite program and impacts of potential usage of hyperspectral IR sounder. 2021 IEEE Int. Geosci. Remote Sens. Symp. IGARSS, Brussels, Belgium, 1507-1510.
[https://doi.org/10.1109/IGARSS47720.2021.9553888]
-
Chahine, M. T., and Coauthors, 2006: AIRS: Improving weather forecasting and providing new data on greenhouse gases. Bull. Amer. Meteor. Soc., 87, 911-926.
[https://doi.org/10.1175/BAMS-87-7-911]
-
Cho, Y.-J., D.-B. Shin, T.-Y. Kwon, J.-C. Ha, and C.-H. Cho, 2014: Retrieval of thermal tropopause height using temperature profile derived from AMSU-A of Aqua satellite and its application. Atmosphere, 24, 523-532.
[https://doi.org/10.14191/Atmos.2014.24.4.523(inKoreanwithEnglishabstract)]
-
Cho, Y.-J., H.-S. Jang, J.-C. Ha, R. K. Y. Choi, K.-H. Kim, E. Lim, J.-H. Yun, J.-I. Lee, and J.-I. Seong, 2017: Estimation of oceanic Total Precipitable Water from HALE UAV. Atmosphere, 27, 359-370.
[https://doi.org/10.14191/Atmos.2017.27.3.359(inKoreanwithEnglishabstract)]
-
Cucurull, L., R. A. Anthes, S. P. F. Casey, M. J. Mueller, and A. Vidal, 2024: Observing System Simulation Experiments (OSSEs) in support of Next-Generation NOAA satellite constellation. Bull. Amer. Meteor. Soc., 105, E884-E904.
[https://doi.org/10.1175/BAMS-D-23-0060.1]
-
Eresmaa, R., 2014: Imager-assisted cloud detection for assimilation of infrared atmospheric sounding interferometer radiances. Quart. J. Roy. Meteor. Soc., 140, 2342-2352.
[https://doi.org/10.1002/qj.2304]
-
Eresmaa, R., J. Letertre-Danczak, C. Lupu, N. Bormann, and A. McNally, 2017: The assimilation of Cross-track infrared sounder radiances at ECMWF. Quart. J. Roy. Meteor. Soc., 143, 3177-3188.
[https://doi.org/10.1002/qj.3171]
-
Fujita, T., K. Okamoto, H. Seko, M. Otsuka, H. Owada, and M. Hayashi, 2023: Mesoscale observing system simulation experiment (OSSE) to evaluate the potential impact from a geostationary hyperspectral infrared sounder. J. Meteor. Soc. Japan, 101, 371-390.
[https://doi.org/10.2151/jmsj.2023-022]
- Han, H.-J., J.-H. Kang, and I.-H. Kwon, 2023: Development of global Observing System Simulation Experiment (OSSE) system using Korea Integrated Model (KIM): Description and preliminary result. AOGS 2023 20th annual meeting, Singapore, AS07-A027.
- Han, W., R. Yin, D. Di, J . Li, J. Wang, X. Shen, and Z. Zhang, 2019: Assimilation of high temporal resolution GIIRS in global 4D-Var. 2019 Joint Satellite Conf., Boston, MA, Amer. Meteor. Soc., 8B. 4, [Available online at https://ams.confex.com/ams/JOINTSATMET/meetingapp.cgi/Paper/359776, ].
-
Hilton, F., N. C. Atkinson, S. J. English, and J. R. Eyre, 2009: Assimilation of IASI at the Met office and assessment of its impact through observing system experiments. Quart. J. Roy. Meteor. Soc., 135, 495-505.
[https://doi.org/10.1002/qj.379]
-
Hocking, J., J. Vidot, P. Brunel, P. Roquet, B. Silveira, E. Turner, and C. Lupu, 2021: A new gas absorption optical depth parameterisation for RTTOV version 13. Geosci. Model Dev., 14, 2899-2915.
[https://doi.org/10.5194/gmd-14-2899-2021]
-
Hoffman, R. N., and R. Atlas, 2016: Future observing system simulation experiments. Bull. Amer. Meteor. Soc., 97, 1601-1616.
[https://doi.org/10.1175/BAMS-D-15-00200.1]
- Hoffman, R. N., S. Malardel, and T. Peevey, 2019: New 14-month forecast available for research. ECMWF Newsletter, 158, Winter 2019, [Available online at https://www.ecmwf.int/en/newsletter/158/news/new-14-month-forecast-availableresearch, ].
-
Holmlund, K., and Coauthors, 2021: Meteosat Third Generation (MTG): continuation and innovation of observations from geostationary orbit. Bull. Amer. Meteor. Soc., 102, E990-E1015.
[https://doi.org/10.1175/BAMS-D-19-0304.1]
-
Hong, S.-Y., and Coauthors, 2018: The Korean Integrated Model (KIM) system for global weather forecasting. Asia-Pac. J. Atmos. Sci., 54, 267-292.
[https://doi.org/10.1007/s13143-018-0028-9]
-
Iturbide-Sanchez, F., Z. Wang, S. Kalluri, Y. Chen, E. Lynch, and M. Divakarla, 2022: Exploration of a future NOAA infrared sounder in geostationary Earth orbit. IEEE J. Sel. Top. Appl. Earth Obs. Remote Sens., 15, 1543-1561.
[https://doi.org/10.1109/JSTARS.2022.3142069]
-
Jones, T. A., S. Koch, and Z. Li, 2020: Assimilating synthetic hyperspectral sounder temperature and humidity retrievals to improve severe weather forecasts. Atmospheric Res., 186, 9-25.
[https://doi.org/10.1016/j.atmosres.2016.11.004]
-
Joo, S ., J. E yre, and R. Marriott, 2013: T he impact o f MetOp and other satellite data within the Met Office global NWP system using an adjoint-based sensitivity method. Mon. Wea. Rev., 186, 3331-3342.
[https://doi.org/10.1175/MWR-D-12-00232.1]
- Kim, H.-S., and J.-H. Kang, 2022a: Development of data preprocessing system for GIIRS/FY-4A. 2022 EUMETSAT Conference, Brussels, Belgium, 19-23 Sep., 2022.
- Kim, H.-S., and J.-H. Kang, 2022b: Development of Assimilation Techniques for the First GEO Hyperspectal IR Sounder (GIIRS/FY-4A). 2022 KIAPS International Symposium-on the Global NWP System Modeling, Seoul, Korea, 14-16 Nov., 2022.
-
Li, J., A. J. Geer, K. Okamoto, J. A. Otkin, Z. Liu, W. Han, and P. Wang, 2021: Satellite All-sky infrared radiance assimilation: Recent progress and future perspectives. Adv. Atmos. Sci., 39, 9-21.
[https://doi.org/10.1007/s00376-021-1088-9]
-
Li, J., A. J, W. P. Menzel, T. J. Schmit, and J. Schmetz, 2022: Applications of geostationary hyperspectral infrared sounder observations: Progress, challenges, and future perspectives. Bull. Amer. Meteor. Soc., 103, E2733-E2755.
[https://doi.org/10.1175/BAMS-D-21-0328.1]
-
McGrath-Spangler, E. L., W. McCarty, N. C. Privé, I. Moradi, B. M. Karpowicz, and J. McCorkel, 2022: Using OSSEs to evaluate the impacts of geostationary infrared sounders. J. Atmos. Oceanic Technol., 39, 1903-1918.
[https://doi.org/10.1175/JTECH-D-22-0033.1]
-
McNally, A. P., and P. D. Watts, 2003: A cloud detection algorithm for high-spectral-resolution infrared sounders. Quart. J. Roy. Meteor. Soc., 129, 3411-3423.
[https://doi.org/10.1256/qj.02.208]
-
Menzel, W. P., T. J. Schmit, P. Zhang, and J. Li, 2018: Satellite-based atmospheric infrared sounder development and applications. Bull. Amer. Meteor. Soc., 99, 583-603.
[https://doi.org/10.1175/BAMS-D-16-0293.1]
- NMSC, 2023: Medium and long-term development plan of National Meteorological Satellite Center. National Meteorological Satellite Center, 74 pp.
-
Noh, Y.-C., H.-L. Huang, and M. D. Goldberg, 2021: Refinement of CrIS channel selection for global data assimilation and its impact on the global weather forecast weather and forecasting. Wea. Forecasting, 36, 1405-1429.
[https://doi.org/10.1175/WAF-D-21-0002.1]
-
Okamoto, K., and Coauthors, 2020: Assessment of the potential impact of a hyperspectral infrared sounder on the Himawari follow-on geostationary satellite. SOLA, 16, 162-168.
[https://doi.org/10.2151/sola.2020-028]
-
Posselt, D. J., L. Wu, M. Schreier, J. Roman, M. Minamide, and B. Lambrigtsen, 2022: Assessing the forecast impact of a geostationary microwave sounder using regional and global OSSEs. Mon. Wea. Rev., 150, 625-645.
[https://doi.org/10.1175/MWR-D-21-0192.1]
-
Strow, L. L., H. Motteler, D. Tobin, H. Revercomb, S. Hannon, H. Buijs, J. Predina, L. Suwinski, and R. Glumb, 2013: Spectral calibration and validation of the Cross-track Infrared Sounder (CrIS) on the Suomi NPP satellite. J. Geophys. Res. Atmos., 118, 12,486-12,496.
[https://doi.org/10.1002/2013JD020480]
- WMO, 2019: Vision f or t he W MO i ntegrated global observing system in 2040. WMO Doc., 1243, World Meteorological Organization, 47 pp.
-
Yang, J., Z. Zhang, C. Wei, F. Lu, and Q. Guo, 2017: Introducing the new generation of Chinese geostationary weather satellites, Fengyun-4. Bull. Amer. Meteor. Soc., 98, 1637-1658.
[https://doi.org/10.1175/BAMS-D-16-0065.1]
-
Zeng, X., and Coauthors, 2020: Use of Observing System Simulation Experiments in the United States. Bull. Amer. Meteor. Soc., 101, E1427-1438.
[https://doi.org/10.1175/bams-d-19-0155.1]