
WRF-UCM을 활용한 수도권 지역의 열환경 변화 연구 : 2000년과 2009년의 비교
Abstract
This study examined the impact of change of land-use and meteorological condition due to urbanization on heat environment in Seoul metropolitan area over a decade (2000 and 2009) using Weather Research and Forecasting (WRF)-Urban Canopy Model (UCM). The numerical simulations consist of three sets: meteorological conditions of (1) October 2000 with land-use data in 2000 (base simulation), (2) October 2009 with land-use data in 2000 (meteorological condition change effect) and (3) October 2009 with land-use data in 2009 (both the effects of land-use and meteorological condition change). According to the experiment results, the change of land-use and meteorological condition by urbanization over a decade showed different contribution to the change of heat environment in Seoul metropolitan area. There was about 1°C increase in near-surface (2 m) temperature over all of the analyzed stations due to meteorological condition change. In stations where the land-use type changed into urban, large temperature increase at nighttime was observed by combined effects of meteorological condition and land-use changes (maximum 4.23°C). Urban heat island (UHI) over 3°C (temperature difference between Seoul and Okcheon) increased 5.24% due to the meteorological condition change and 26.61% due to the land-use change. That is, land-use change turned out to be contributing to the strengthening of UHI more than the meteorological condition change. Moreover, the land-use change plays a major role in the increase of sensible heat flux and decrease of latent heat flux.
Keywords:
Urban canopy model, urbanization, urban heat island, land-use, heat flux1. 서 론
도시의 인구 집중에 따라 도시 내 기상 및 기후에 대한 관심이 증가하고 있다. 이는 거대한 도시를 형성하면서 도시열섬, 강수 증가, 바람길 등 도시만의 독특한 기후를 형성하기 때문이다(Kim et al., 2011). 도시화로 인한 가장 큰 변화는 인공지표의 증가와 함께 고층 건물의 증가이다. 인공지표는 다른 지표에 비해 열용량이 크고 수분함량이 적으며 알베도가 작다(NCAR, 2005; Koo et al., 2007; Lee et al., 2008). 또한 도시의 건물들은 거칠기 길이를 증가시키고 바람길 형성에 영향을 끼친다(Bornstein, 2001; Hunt et al., 2003). 도시의 높은 건물들은 지표에 그늘효과를 내어 표면을 차게 만드는 반면 단파와 장파 복사를 가두는 역할을 하여 기온을 증가시키기도 한다(Kusaka et al., 2001).
따라서 수도권 지역의 도시화에 따른 토지피복의 변화가 국지 기상에 어떠한 영향을 주는지 중규모 수치모형을 이용하여 분석한 연구들이 많이 진행되어 왔다(Moon and Koo, 2006; Jeong and Kim, 2008; Kang et al., 2010; Pathirana et al., 2014). Moon and Koo (2006)와 Kang et al. (2010)은 수도권 지역을 대상으로 U. S. Geological Survey (USGS)의 1990년대 토지피복지도와 환경부의 2000년대 토지피복지도를 이용하여 도시화에 따른 토지피복변화가 국지기상에 미치는 영향을 수치 모델링을 통해 분석하였다. 이를 통해 도시화가 나타난 지역에 기온이 상승하여 도시 열섬현상을 가중시키고 풍속이 감소함을 밝혔다. 토지피복의 변화는 기온과 바람뿐만 아니라 강수에도 크게 영향을 미친 것으로 분석되었다(Pathirana et al., 2014). 4개의 호우사례에 대하여 실제 토지피복지도와 이를 임의로 2배 증가시킨 토지피복지도를 사용하여 수치 실험을 수행한 결과, 도시가 발달한 지역의 강우 강도가 뚜렷하게 증가함을 보였다. 이들은 공통적으로 도시화로 인한 토지피복 변화가 도시 규모의 기상 및 기후에 미치는 영향이 크다는 것을 제시하였다. 그러나 선행 연구들은 단일 도시항목을 포함하는 토지피복지도를 다루고 있다는 한계점이 있다. 도시가 다양한 형태로 발전하고 고층 건물이 증가하면서 도시지역의 기상현상은 더욱 복잡해지고 있다. 따라서 최근에는 도시 빌딩 및 협곡을 재현한 도시캐노피모형(Urban Canopy Model, 이하 UCM)을 중규모 모형에 결합한 후 도시의 기상 현상을 다루고 있는 추세이다(Jiang et al., 2008; Wang et al., 2009a, b; Grossman-Clarke et al., 2010). UCM은 빌딩의 지붕, 벽면, 도로 사이의 에너지 교환을 고려하여 도시의 복잡한 물리 과정을 포함하고 단일 도시항목을 세 개의 도시항목(저밀도주거지역, 고밀도주거지역, 상업/산업/교통지역)으로 세분화하여 다룰 수 있는 장점이 있다.
Weather Research and Forecasting (WRF)-UCM은 도시지역 기상현상 중 특히 열환경 모의에서 높은 정확도를 보여 왔기 때문에 이 모델을 활용한 연구들은 주로 도시의 폭염 및 열섬현상에 초점을 두었다(Lin et al., 2008; Grossman-Clarke et al., 2010; Meng et al., 2011; Lee et al., 2012; Chen et al., 2014). Grossman-Clarke et al. (2010)은 미국 피닉스 지역의 폭염사례를 선정한 후 1973년, 1985년, 1998년, 2005년의 지표이용도를 대상으로 WRF-UCM 기반 수치실험을 수행하였다. 그 결과, 도시의 발달이 극한 기온을 증가시켰으며 그 효과는 주간보다 야간에 뚜렷하였다. 또한 도시가 발달함에 따라 그 지역의 바람이 약화되었으며 한랭이류가 감소하였다. 한편, 열환경뿐만 아니라 도시화로 인해 야기되는 에어로졸과 오존의 변화를 중점적으로 살펴본 연구들도 있었다(Jiang et al., 2008; Wang et al., 2009a, b). Jiang et al. (2008)는 50년 후(2000년대와 2050년대)의 오존 농도를 토지피복에 의한 변화와 기후에 의한 변화로 분리하여 분석함으로써 선행 연구와 차별성을 보였다.
따라서 국내 수도권지역의 열환경 변화에서도 토지피복변화 효과뿐만 아니라 기후 또는 기상환경 변화에 의해 발생하는 효과를 함께 고려함으로써 비교 분석할 필요가 있다. 이러한 연구는 기후 또는 기상환경 변화에 비하여 토지피복 변화가 도시의 열환경 변화에 얼마나 기여하는가를 정량적으로 제시할 수 있다. 그러므로 본 연구는 수도권 지역을 대상으로 10년 사이(2000년과 2009년) 도시화로 인한 토지피복변화와 기상환경변화가 열환경에 미치는 영향을 WRFUCM을 통해 살펴보았다. 2장에서는 본 연구에서 사용한 수치모델, 자료, 실험설계 등 실험방법에 대해 설명하였으며 3장에서는 10년 후의 토지피복 및 열환경 변화에 대한 주요 분석 결과를 제시하고 난 후 결과를 요약하고 논의하였다.
2. 실험방법
2.1 수치모델
본 연구에서 수도권 지역의 열환경을 모의하기 위해 도시캐노피모델(Urban Canopy Model, UCM)이 결합된 Weather Research and Forecasting (WRF, version 3.5) 모델을 사용하였다. Figure 1은 Kusaka et al. (2001)과 Kusaka and Kimura (2004)의 연구에서 개발된 단일 층 UCM의 구조를 보여준다. 단일 층 UCM은 유한한 높이를 가진 빌딩이 도시 협곡을 만드는 구조이고 태양 방위각의 일 변화를 포함하여 복사가 고려된다. 협곡 내에서는 빌딩의 그늘효과와 복사의 재반사 효과를 고려하여 빌딩의 옥상, 벽면, 도로에 대한 표면온도, 플럭스(현열, 잠열, 운동량), 기온 및 바람의 연직 프로파일을 추정하는 등 도시의 복잡한 물리 과정을 포함하고 있다. 또한 본 연구에서는 도시가 아닌 지면의 물리과정을 표현하기 위해 지면모델인 Noah land surface model (Mitchell, 2000)이 함께 사용되었다.
2.2 자료
WRF에서 기본적으로 제공되는 지표이용도는 U. S. Geological Survey (USGS)이다. 그러나 이 지도는 1990년 초반에 제작된 것으로 최근 한국 수도권 지역의 도시화를 반영하지 못하고 있다. 따라서 본 연구는 USGS의 수도권 지역(서울, 인천, 경기 지역) 지표이용도를 환경부 환경공간정보서비스(http://egsi.me.go.kr)에서 제공하는 국내 토지피복지도 중 중분류 자료로 대체하였다. 중분류는 해상도가 5 m이고 23개의 항목으로 분류되어 있어 24개로 분류된 USGS와 동시에 사용하기 적합하다. 수도권 지역의 토지피복지도는 구축시기에 따라 세 가지로 분류될 수 있으며 2000년과 2007년에 구축된 지도와 2009년에 갱신된 지도로 구성된다. 토지피복변화와 기상환경변화에 의해 야기되는 도시의 열환경 변화를 명백히 살펴보기 위해 2000년과 2009년의 토지피복지도를 선택하여 수치실험에 활용하였다. 본 토지피복지도는 USGS의 토지피복 항목에 적합하게 재분류되었다. 또한 UCM 적용을 위해 토지피복지도 내의 단일 도시항목을 저밀도주거지역, 고밀도주거지역, 상업/산업/교통지역의 세 가지 도시 하위항목으로 세분화하였다. 환경부 토지피복지도의 주거지역 분류는 통계청 통계지리정보서비스(http://sgis.kostat.go.kr)에서 제공되는 수도권 지역의 2000년과 2009년 인구밀도 자료를 사용하여 구분하였다.
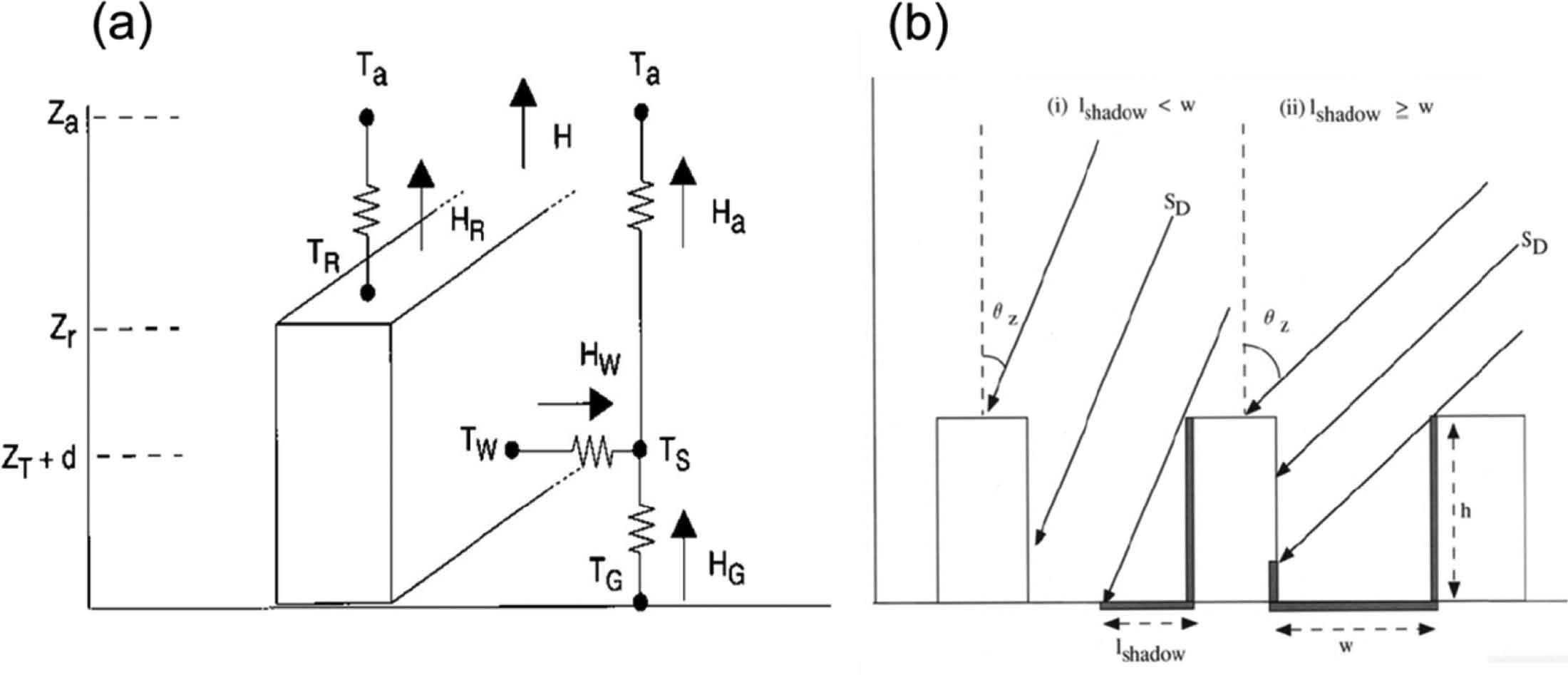
(a) Schematic of the single-layer urban canopy model: Ta is the air temperature at reference height Za, TR is the building roof temperature, TW is the building wall temperature, TG is the road temperature, and TS is the temperature defined at ZT + d. H is the sensible heat exchange at the reference height. Ha is the sensible heat flux from the canyon space to the atmosphere. HW is that from wall to the canyon space. HG is that from road to the canyon space and HR is that from roof to the atmosphere. (b) The direct solar radiation (SD) incident on a horizontal surface. w is the normalized road width, h is the normalized building height (w + r = 1). Here r is the normalized roof width. lshadow is the normalized shadow length on the road θz is solar zenith angle (From Kusaka and Kimura, 2004).
2000년과 2009년 10월의 기상환경을 살펴보기 위해 본 연구에서는 수도권 지역에 위치한 30개 지점의 일 강수량 자료와 National Centers for Environmental Prediction and National Center for Atmospheric Research (NCEP/NCAR) 재분석 자료의 1000 hPa 지위고도와 기온자료를 사용하였다. 또한 모델 평가를 위해 동일한 기간에 해당하는 서울(108), 인천(112), 수원(119)의 시간별 기온자료를 사용하였다.
2.3 실험설계
실험 기간은 본 연구에서 선택된 토지피복지도와 동일한 해인 2000년과 2009년의 10월 1일부터 31일로 선정하였다. Figure 2는 2000년과 2009년 10월의 평균적인 기상장이다. 두 해 모두 한반도는 대륙 고기압의 영향을 받고 있고 2009년보다 2000년에 대륙 고기압이 한반도 쪽으로 더 많이 확장하였다. 기온장은 두 해 모두 유사한 형태를 보였으나 한반도를 지나는 15°C의 기온선이 2000년보다 2009년에 고위도에 위치하여 2009년이 상대적으로 높은 기온을 보였다. 수도권지역의 10월 총 강수량 분포를 통해(Fig. 3), 2000년(10.5~26.5 mm)에 비해 2009년(53.0~96.5 mm) 10월이 다습한 달임을 알 수 있었다.
본 연구는 수도권 지역의 10년 후 열환경 변화를 토지피복변화 효과와 기상환경변화 효과로 분리하기 위해 총 세 개의 실험을 구성하였다(Table 1). 첫 번째 실험은 기본 실험으로써 2000년 토지피복지도를 이용하여 2000년 10월의 기상환경을 모의한다(LU00). 두 번째 실험은 2000년 토지피복지도를 이용하여 2009년 10월의 기상환경을 모의한다(Meteorological condition change effect, MC09). 이 실험은 토지피복지도가 변하지 않고 고정되어 있기 때문에 2000년과 2009년 사이의 기상환경변화만을 고려하는 것으로 간주한다. 세 번째 실험은 2009년 토지피복지도를 이용하여 2009년 10월의 기상환경을 모의한다(Meteorological condition과 Land use change effect, MC-LU09). 이 실험은 토지피복지도와 기상환경이 모두 달라졌기 때문에 토지피복변화와 기상환경변화를 동시에 고려하는 것으로 간주한다. 따라서 세 번째 실험(MC-LU09)과 두 번째 실험(MC09)의 결과 차이는 토지피복변화만을 고려하는 것으로 간주한다.
실험 도메인은 27 km, 9 km, 3 km, 1 km의 수평해상도(Fig. 4a)와 27개의 연직층을 가지고 있다. 본 연구에서는 마지막 도메인(Fig. 4b)의 10월 1일부터 31일까지의 1시간 간격의 모의 결과를 사용하였다. 지형자료는 USGS earth resources observation systems data center에 의해 제작된 전지구 지형자료인 Global 30 arc-second elevation (GTOPO30) 자료와 USA National Geospatial-Intelligence Agency (NGA), National Aeronautics and Space Administration (NASA), the Italian Space Agency (ASI), German Aerospace Center (DLR)에서 공동 제작한 한반도 영역의 Shuttle Radar Topography Mission (SRTM) 3초 자료를 사용하였다. 모형의 초기 및 경계 조건은 NCEP final analysis (FNL) 자료를 사용하였으며 이 자료는 1° × 1° 해상도를 가지고 6시간 간격으로 제공된다. 경계층 물리화과정 모수화는 YSU 방법을, 미세 물리 모수화 방법은 WRF double-moment 6-class graupel 방법을, 장파복사와 단파복사는 각각 Rapid radiative transfer Model 방법, Dudhia 방법을 사용하였다. 적운 모수화는 Kain-Fritsch 방법을 사용하였으며 마지막 도메인에 대해서는 적용하지 않았다(Table 2). WRF-UCM를 구동하기 위해 입력되는 도시 변수들은 Table 3에 제시하였으며 모든 실험에 동일하게 적용하였다.
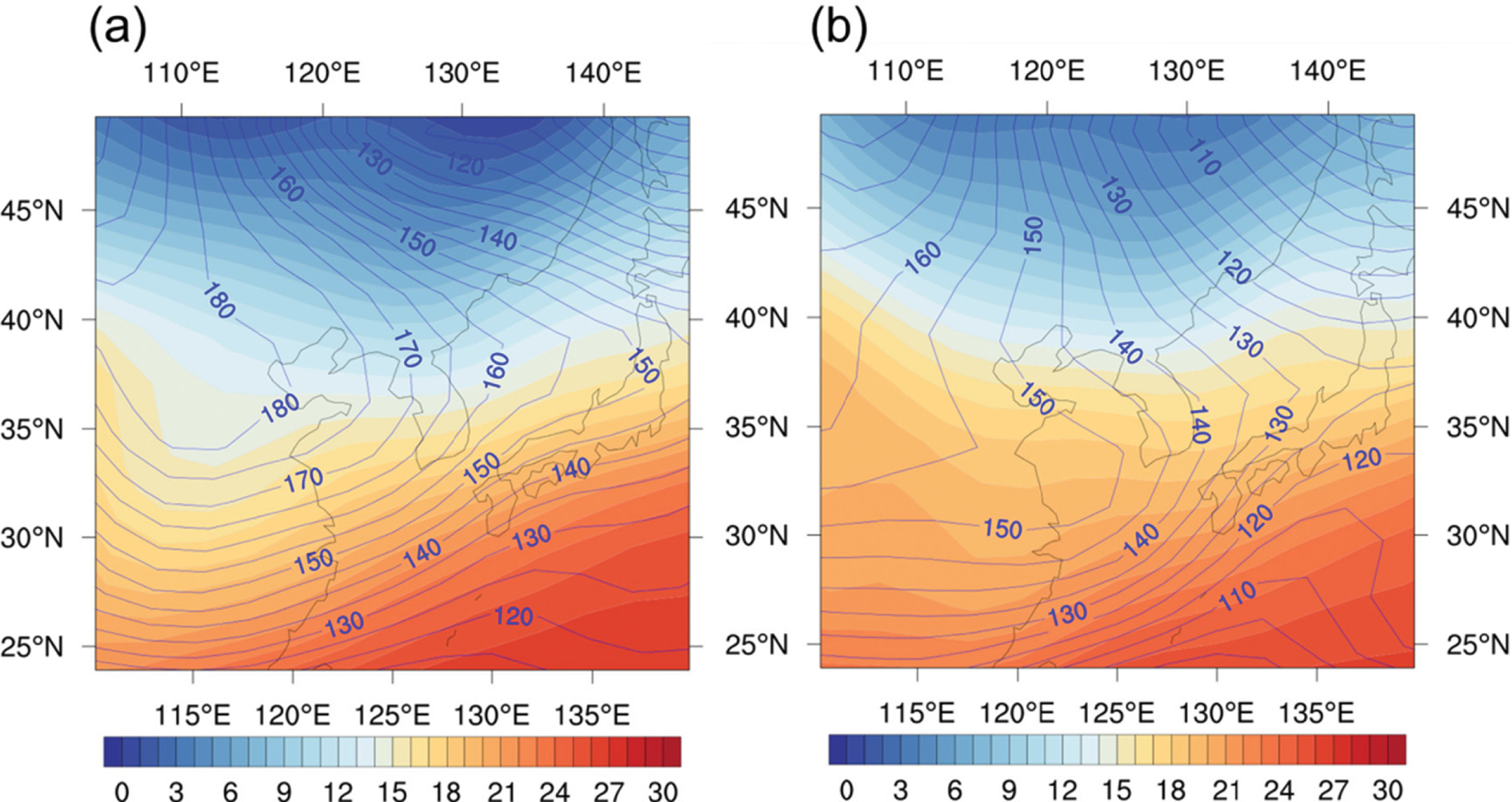
Synoptic distributions averaged over October in (a) 2000 and (b) 2009 showing geopotential height (solid blue line) and air temperature (shaded) at 1000 hPa.
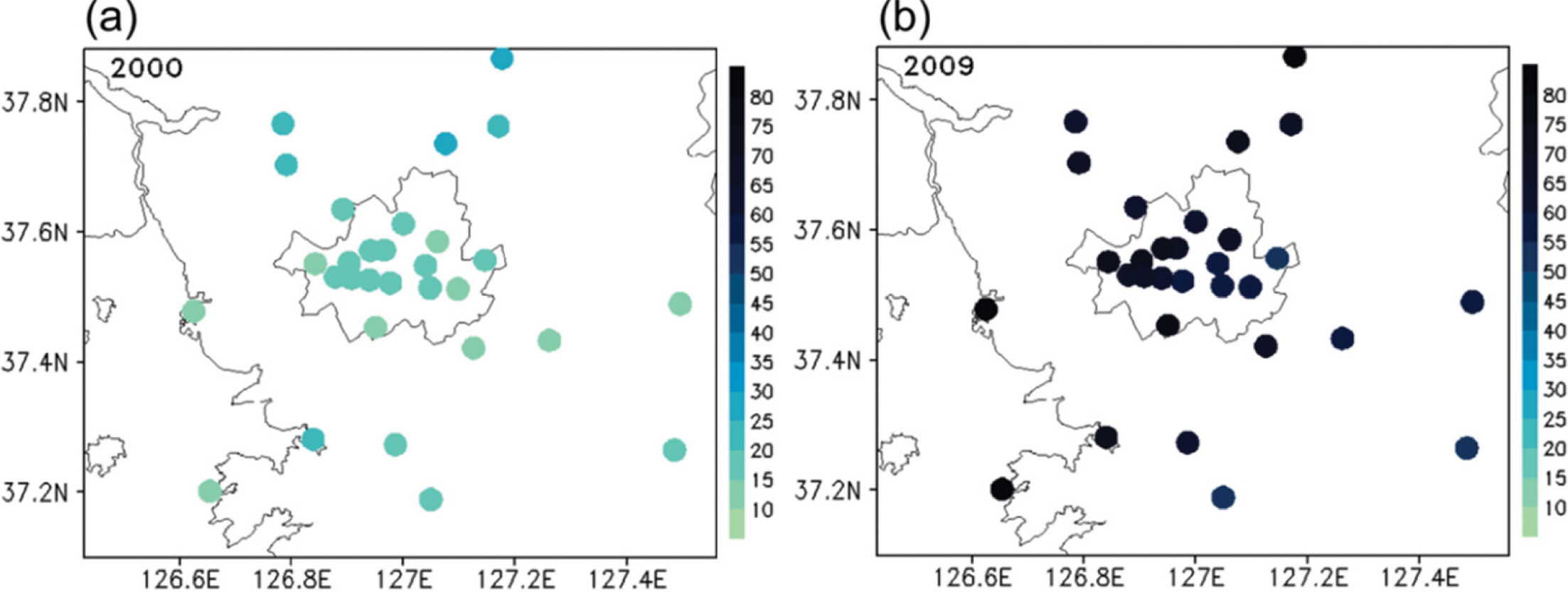
Distribution of monthly total precipitation (unit: mm) at 30 stations over Seoul metropolitan area during October in (a) 2000 and (b) 2009.
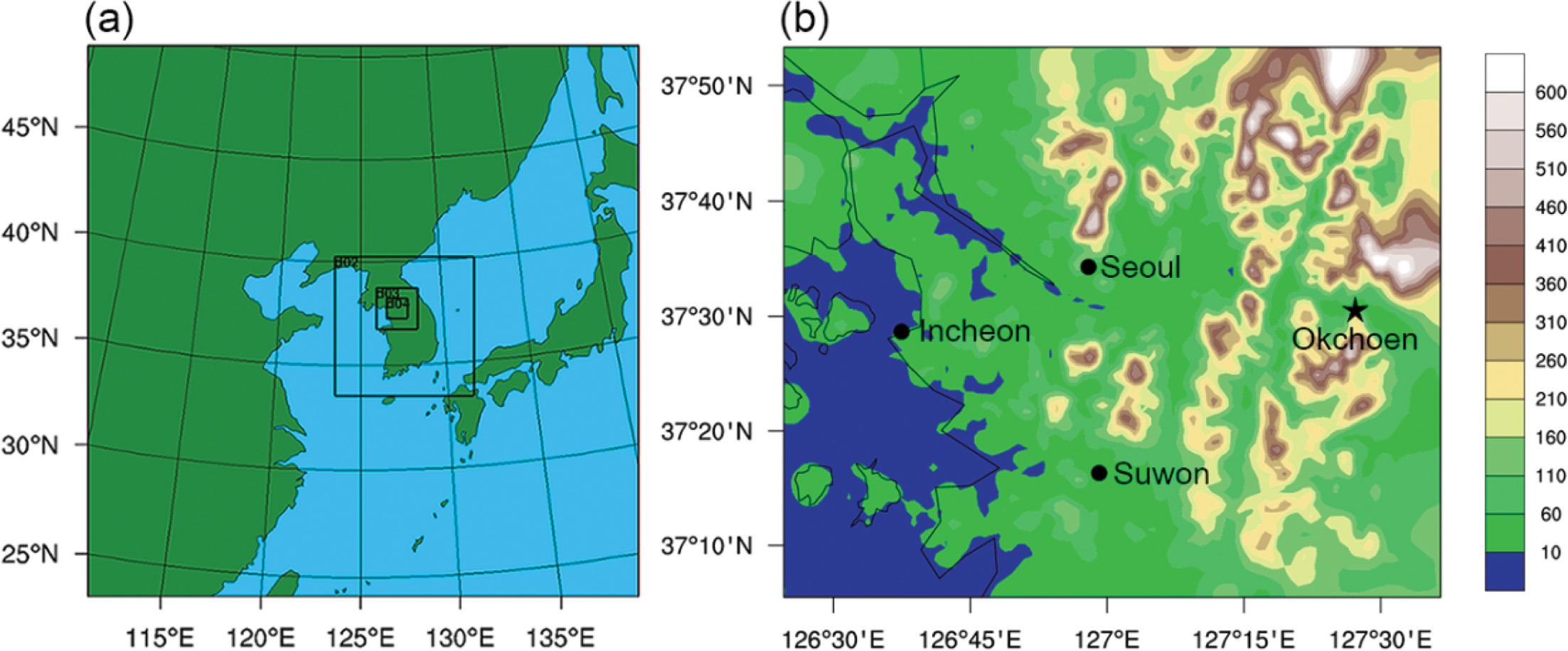
(a) Configuration of nested model domains. (b) The finest domain. Topography is shaded with intervals of 50 m. The location of weather stations for validation (dots) and analysis (star) are shown.
2.4 모델 평가
먼저 WRF-UCM 수행 결과의 신뢰성을 얻기 위해 모델의 평가가 필요하다. 3개의 실험 중 입력자료(토지피복지도, 기상환경)의 연도가 동일한 LU00와 MC-LU09의 실험 결과를 관측치와 비교하고 통계검증을 수행하였다. 평가 지점은 서울/경인지역의 대표 지점인 서울, 인천, 수원이다. Figure 5는 지점 평균된 2000년(LU00)과 2009년(MC-LU09) 10월 1일부터 31일까지 관측값과 모의값의 2 m 기온시계열이다. 2000년과 2009년의 WRF-UCM 모의값은 그 기간 동안의 기온의 일 변화 경향을 잘 모의하는 것으로 판단된다. 2000년과 2009년의 평가 지점 기온 오차를 박스플롯으로 나타내었고(Fig. 6) 통계검증 결과를 Table 4에 제시하였다. 모의값은 2000년의 경우 두 지점(서울, 인천)에서 관측값을 과소평가하고 2009년의 경우 모든 지점에서 관측값을 과대평가하였다. 그러나 Mean Bias Error (MBE)가 평균적으로 0.73°C로 관측값과 모의값 사이의 좋은 일치도를 보여준다. 또한 RMSE는 모든 지점에서 2°C 내외로 나타나고 IOA 또한 2000년과 2009년 세 지점 모두 0.9 이상으로 WRF-UCM 모의값을 신뢰할 수 있을 것으로 판단된다.
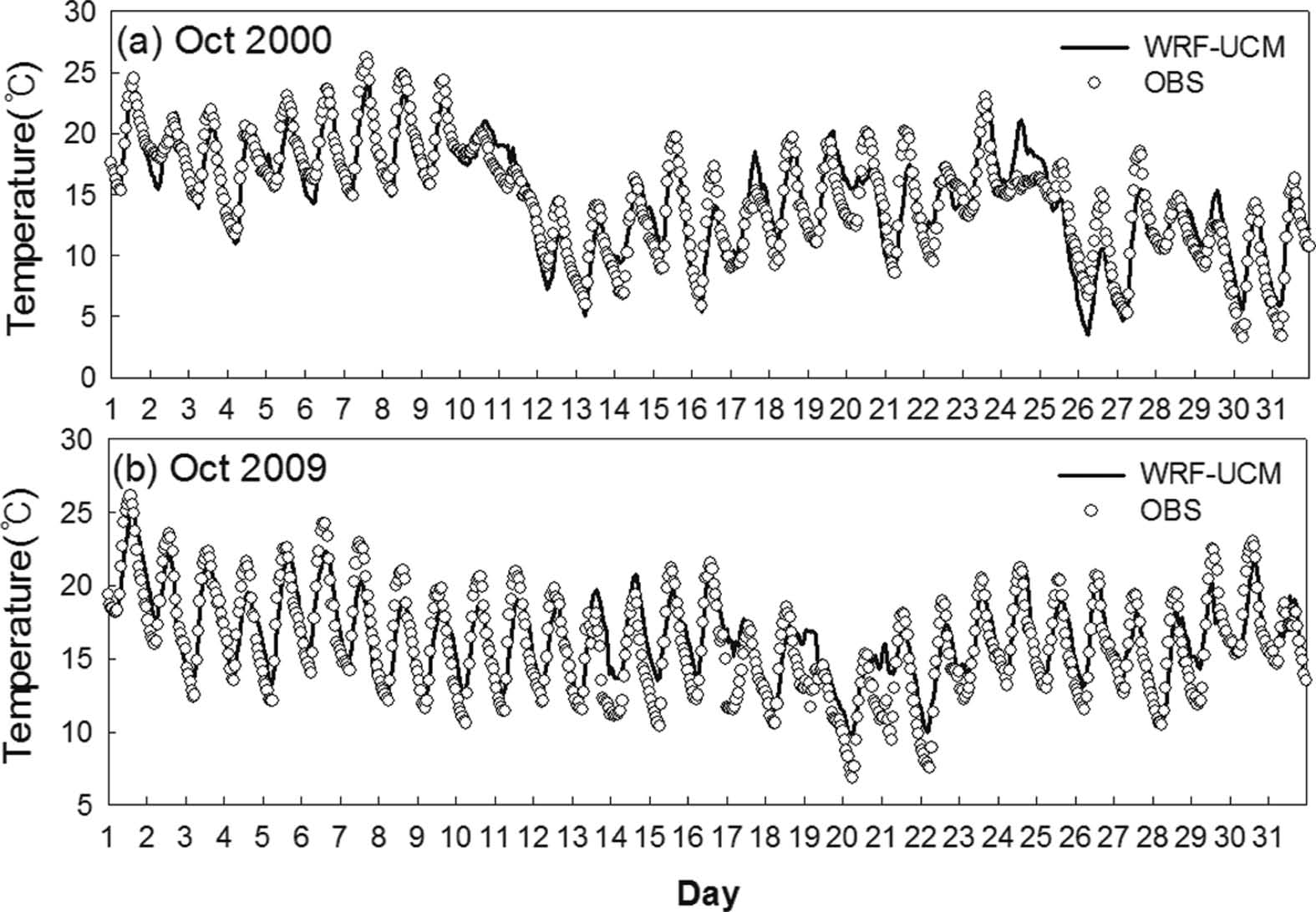
Time series of hourly 2 m temperature averaged for three weather stations from 1 to 31 October, (a) 2000 and (b) 2009. The solid lines and white dots denote observed and simulated values, respectively.
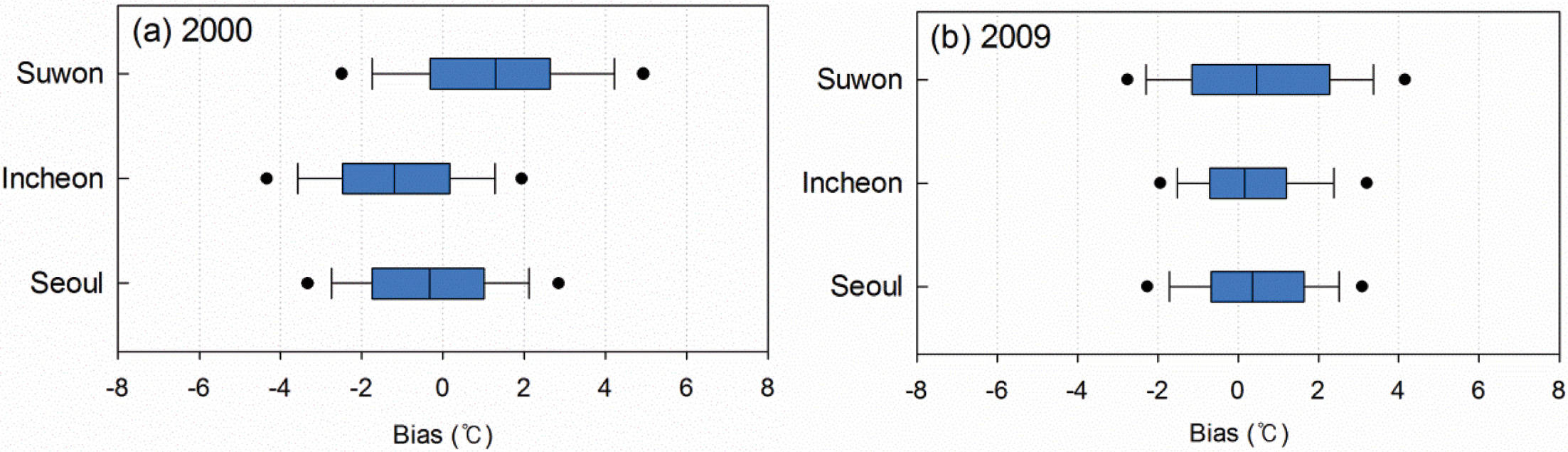
Box plots of bias calculated with the instantaneous 2 m temperature of WRF-UCM and observation data over Seoul, Incheon, and Suwon in (a) 2000 and (b) 2009. The plots show 5th, 10th, 25th, median, 75th, 90th, and 95th percentiles.
3. 결과 및 토의
3.1 토지피복의 변화
Table 5는 실험 도메인에 대한 2000년과 2009년의 토지피복 항목의 비율을 나타낸 것이다. 2000년과 2009년의 실험 도메인에서 도시항목으로 분류된 격자의 비율은 각각 12.52%, 14.70%로 10년 사이 수도권 지역은 다소 약한 도시 성장을 이루었음을 알 수 있다(2.18%). 그러나 도시 하위항목별로 살펴보면, 저밀도/고밀도주거지역의 비율이 감소한(−0.84%) 반면, 상업/산업/교통지역의 비율이 증가한(+3.44%) 것을 볼 수 있다. 특히 상업/산업/교통지역의 비율 변화는 여러 토지피복 항목 중 가장 크게 나타났다. 이는 수도권 지역의 도시 성장뿐만 아니라 도시 특성의 변화가 있었음을 나타낸다. Figure 7은 2000년과 2009년의 실험 도메인에서 도시 하위항목의 공간분포이다. 수도권 지역은 1900년대 후반 급격히 도시화가 진행되어 2000년에 이미 서울을 중심으로 도시가 많이 발달되어 있음을 알 수 있다. 수도권 중심 영역(Fig. 7 실선 영역)의 경우, 도시항목의 비율이 2000년에는 51.33%이고 2009년에는 50.69%로 오히려 0.64% 감소하였다. 그러나 도시 하위항목별로 살펴보면 저밀도주거지역이 6.94%에서 5.12%로, 고밀도주거지역이 29.46%에서 20.81%로 감소하였고 상업/산업/교통지역은 14.94%에서 24.77%로 증가하였다. 즉, 수도권 중심 영역에서 기존의 주거지역이 상업/산업/교통지역으로 도시 특성이 변화되었음을 알 수 있다. 도시 발달이 서울외곽으로 분산되면서 수도권 주변지역에서는 산발적으로 도시가 성장하였다.
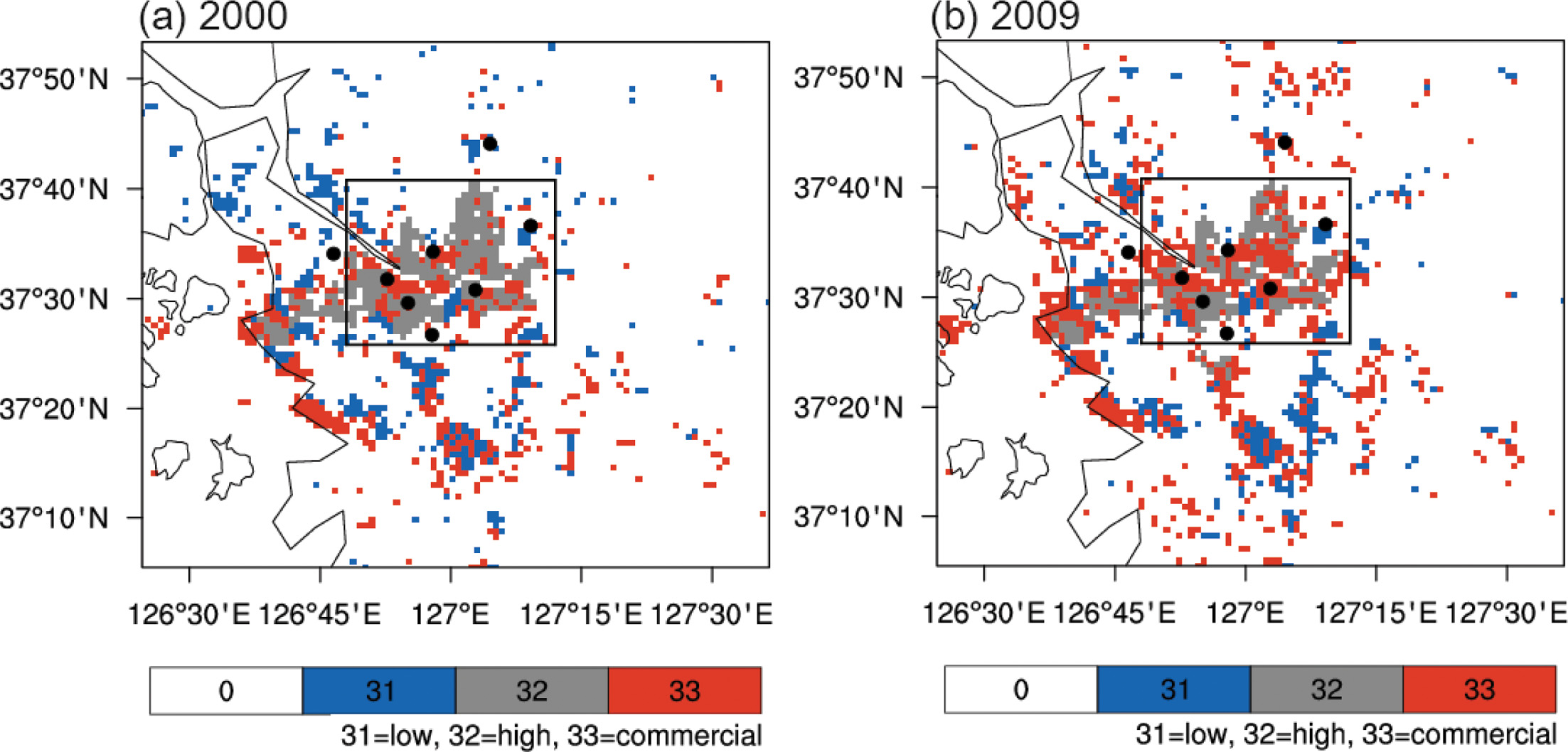
The spatial distributions of urban land-use categories in (a) 2000 and (b) 2009. The blue, gray and red colors denote the low residential, the high residential and the commercial/industrial/transportation, respectively. The core urban area (box) and the location of weather stations for analysis (dots) are shown.
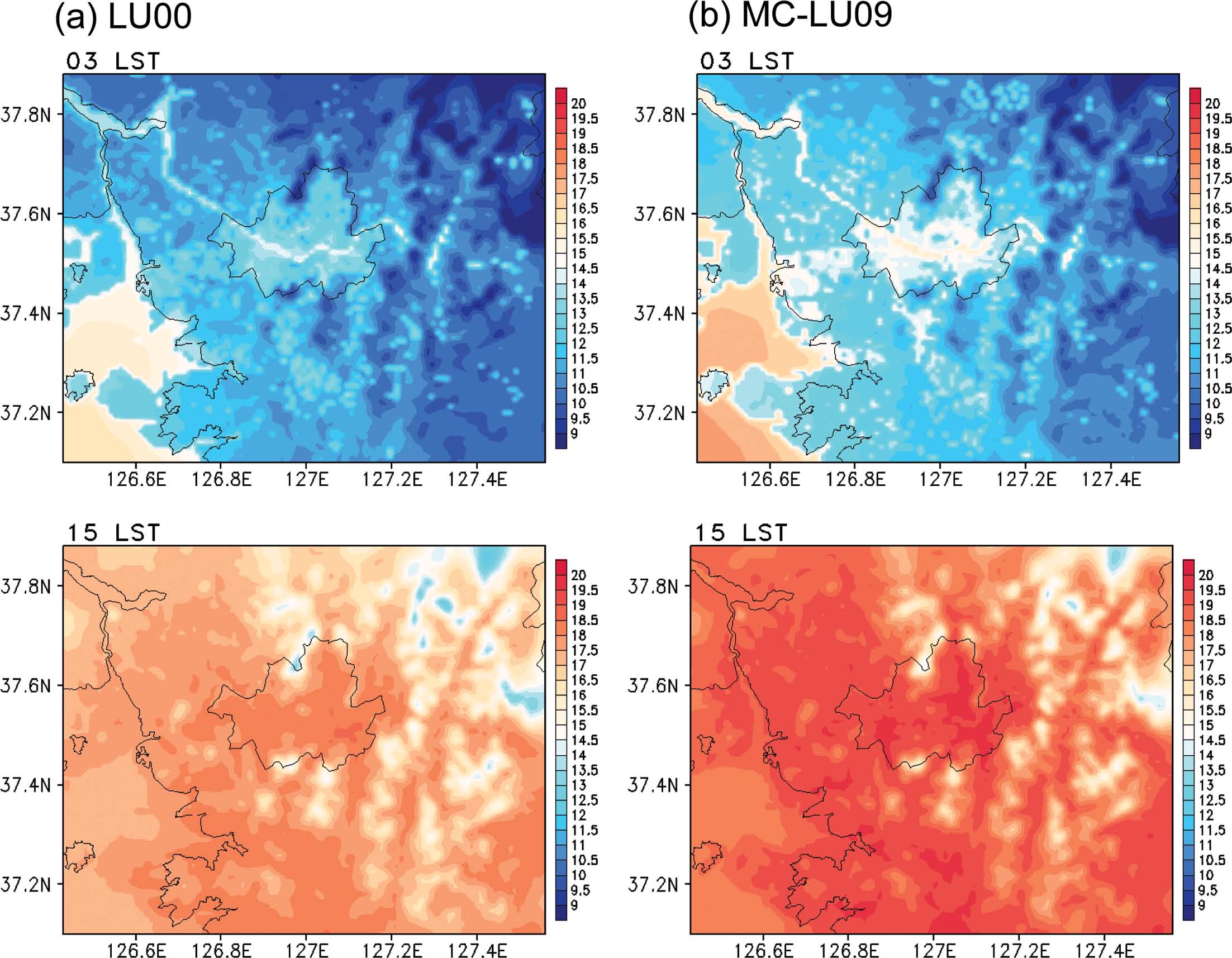
Spatial distributions of simulated 2 m temperature at 0300 LST (upper panels) and 1500 LST (lower panels) averaged during October. (a) LU00 and (b) MC-LU09.
3.2 열환경의 변화
Figure 8는 2000년(LU00)과 2009년(MC-LU09)의 10월 평균 03시와 15시의 2 m 기온 공간분포이다. 모든 시간대에서 서울을 중심으로 고온역이 존재하고 있다. 2000년에 비해 2009년에 전체적으로 높은 기온 분포를 보이면서 10년 사이 기온이 증가하였음을 볼 수있다. 2009년과 2000년의 기온 편차(MC-LU09 minus LU00)의 공간분포를 Fig. 9a에 나타내었다. 03시에는 대부분 지역에서 양의 값을 보이나 음의 값을 보인 지역도 일부 존재하고 있다. 반면에 15시에는 모든 지역에서 양의 값을 보였다. 10년 후의 기온 변화를 토지피복변화 효과(MC-LU09 minus MC09)와 기상환경 변화 효과(MC-LU09 minus LU00)로 분리하여 살펴보았다(Figs. 9b와 9c). 03시의 경우, 기상환경변화로 인해 대부분 지역에서 기온이 증가한 반면 토지피복 변화로 인해 불규칙적으로 기온이 증가한 지역과 감소한 지역이 나타났다. 기온 변화폭은 기상환경변화보다 토지피복변화 효과에서 더 크게 나타났다. 15시는 03시와 유사한 기온분포를 보였으나 기온 변화폭에서 차이를 보였다. 15시에는 기상환경변화로 인해 모든 지역에서 기온이 증가한 반면 토지피복변화로 인해 기온이 증가한 지역도 있고 감소한 지역도 있다. 15시의 기온 변화폭은 03시와는 다르게 기상환경변화보다 토지피복변화 효과에서 더 작게 나타났다.
Figure 10은 도시 특성이 변한 수도권 중심 영역(Fig. 7 실선영역)을 평균한 2000년과 2009년의 2 m 기온의 일 변화이다. 일 변화에서도 10년 후 기온이 증가하였음(0.93~1.53°C)을 볼 수 있다. 이러한 기온 증가를 토지피복변화 효과와 기상환경변화 효과로 분리하여 살펴보았다. 토지피복변화로 인한 기온 상승은 0.07°C에서 0.22°C인 반면 기상환경변화로 인한 기온 상승은 0.78°C에서 1.44°C로 토지피복변화보다 기상환경변화로 인한 기온 상승이 크다. 일 중 가장 큰 기온 증가는 토지피복변화에 의해 23시(0.22°C)에 나타나고 기상환경변화에 의해 10시(1.44°C)에 나타났다.
기온의 일 변화에서 나타나는 토지피복변화 효과와 기상환경변화 효과를 더 명확하게 살펴보기 위하여 각 지점별로 살펴보았다(Fig. 11). 지점은 농경지/수림 지역이 도시지역으로 변한 2개의 지점(남양주시, 의정부시), 주거지역이 상업/산업/교통지역으로 변한 2개의 지점(종로구, 동작구), 토지피복이 변하지 않은 4개의 지점(강서구, 과천시, 강남구, 양천구)이 선정되었다. 지점의 위치는 Fig. 7에 제시되어 있다. 영역 평균(Fig. 10)과 유사하게 지점별에서도 10년 후 기온 증가가 나타났다. 분석한 모든 지점에서 공통적으로 기상환경변화로 인한 기온 증가가 모든 시간대에서 1°C 내외로 나타났다. 기상환경변화에 의한 가장 큰 기온 증가는 낮 시간대(1000~1300 LST)에 나타났다. 반면 토지피복변화에 의한 가장 큰 기온 증가는 늦은 오후와 야간 사이(1800~0200 LST) 또는 이른 아침(0700 LST)에 발생하였다. 따라서 토지피복이 변한 지점(Figs. 11a~d)은 야간 동안 기상환경변화에 의한 기온 증가에 토지피복변화에 의한 기온 증가가 더해져 높은 기온 증가를 보였다. 예를 들어 수림지역이 고밀도주거지역으로 변한 의정부시(532)는 18시부터 다음 날 07시까지 토지피복변화로 인해 평균 2.94°C의 기온 증가를 보였다. 특히 07시에는 기온이 토지피복 변화에 의해 3.35°C, 기상환경변화에 의해 0.88°C 증가하여 10년 후 최대 4.23°C의 기온 증가가 나타났다. 토지피복이 변하지 않은 지점(Figs. 11e~h)의 경우 토지피복변화에 의한 기온 증가나 감소가 적고 기상환경변화에 의한 기온 증가가 대부분을 차지하였다. 따라서 모든 분석 지점에서 기상환경변화 효과로 인한 기온 증가가 모든 시간대에 나타났고 토지피복이 도시지역으로 변화된 지점에서는 토지피복변화 효과가 더해지면서 야간 동안 높은 기온 증가를 보였음을 알 수 있었다.
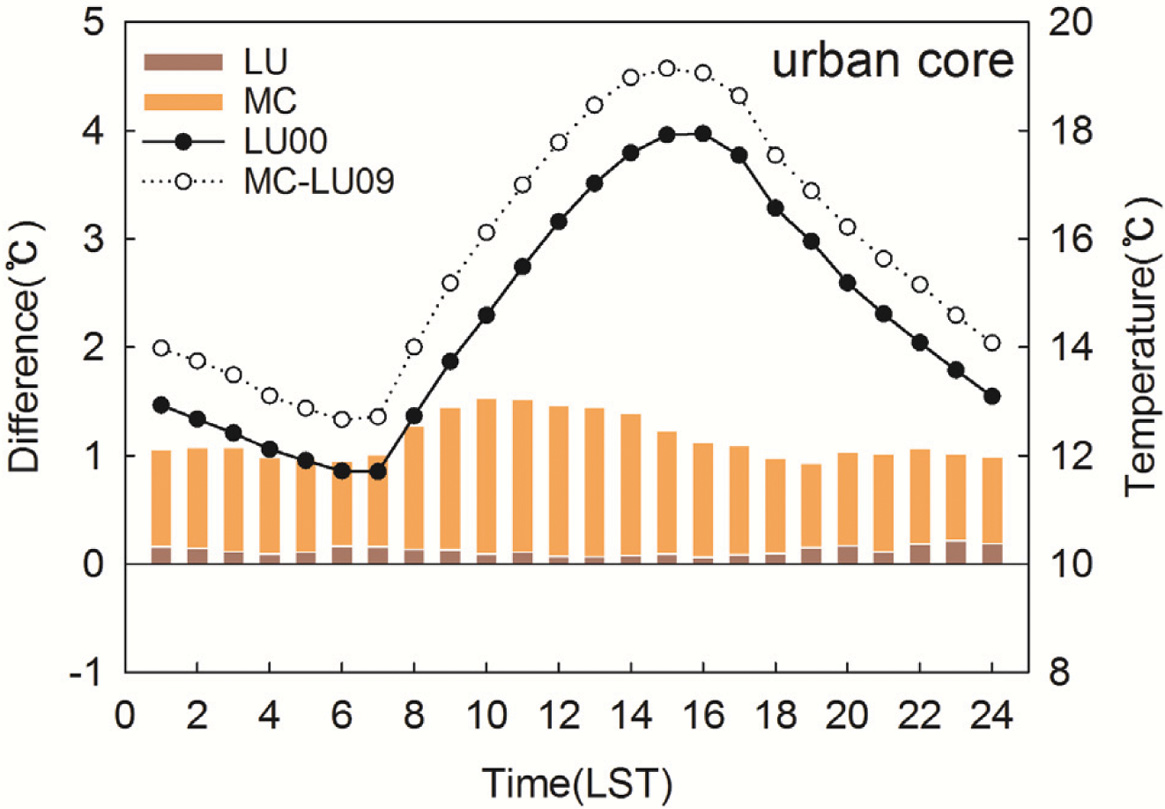
The diurnal variations of 2 m temperature averaged over the core urban area (shown as box in Fig. 7) during October for LU00 (black dots and solid line) and MC-LU09 (white dots and dotted line). The brown and yellow bars denote the temperature increase by land-use change and meteorological condition change, respectively.
도시열섬강도는 도심지역과 교외지역의 기온차로 평가될 수 있다. 본 연구에서는 도시지역으로 분류된 서울(108: 37.57°N, 126.97°E)과 이 지점과 비슷한 위도 대에 위치해 있고 초원/관목지로 분류된 옥천(449: 37.50°N, 127.45°E) 간의 2 m 기온차를 도시열섬강도로 정의하였다(Fig. 4에서 두 지점의 위치 참고). Figure 12는 2000년과 2009년 10월 동안 서울과 옥천 간의 시간 별 2 m 기온차(도시열섬강도)의 빈도 분포이다. 2000년과 2009년의 도시열섬강도는 −4~8°C 범위로 빈도 분포가 양의 값으로 편향되어 있다. 또한 음의 값이 나타나는 것으로 보아 도시열섬현상이 나타나지 않은 시간대도 있음을 알 수 있다. 2000년의 구간별 빈도수는 0~1°C에서 가장 높았고(25.40%) 2009년의 구간별 빈도수는 3~4°C에서 가장 높았다(20.83%). 10년 사이 음의 값의 빈도와 낮은 양의 값(0~2°C)의 빈도가 감소하고 높은 양의 값(2~8°C)의 빈도가 증가하였다. 특히, 2000년과 달리 2009년에는 6°C 이상의 강한 열섬현상이 나타났다. 즉, 이것은 10년 후 도시열섬강도가 증가함을 뜻한다. 10년 후의 도시열섬강도(서울과 옥천의 2 m 기온차)의 변화를 토지피복변화 효과와 기상환경변화 효과로 분리하였다(Fig. 13). 10년 사이 토지피복변화는 1~2°C의 빈도를 가장 크게 감소시키고(−11.82%), 3~4°C의 빈도를 가장 크게 증가시키는데(+9.27%) 기여하였다. 기상환경변화는 −2~−1°C의 빈도를 가장 크게 감소시키고(−5.24%), 2~3°C의 빈도를 가장 크게 증가시키는데(+7.12%) 기여하였다. 3°C 이상의 도시열섬강도는 기상환경변화에 의해 5.24% 증가하고 토지피복변화에 의해 26.61% 증가하였다. 이것은 토지피복변화와 기상환경변화 모두 도시열섬강도를 강화시키는 데 기여함을 의미하고 기상환경변화보다 토지피복변화가 도시열섬강도 강화에 더 큰 영향을 미친다는 것을 시사한다.
Figure 12에서 제시한 빈도 분포를 주간(0700~1800 LST)과 야간(1900~2400 LST, 0100~0600 LST)으로 나누어 분석하였다(Fig. 14). 주간에는 도시열섬강도가 −4~7°C 범위로 양의 값의 빈도가 높으며 2000년과 2009년의 빈도 분포가 유사한 형태를 보였다. 야간은 주간과 달리 2000년과 2009년의 빈도분포에 큰 차이를 보였다. 2000년에는 1~2°C 구간에서 최대의 빈도(15.32%)를 보이고 2009년에는 3~4°C 구간에서 최대의 빈도(15.59%)를 보이며 10년 후 빈도 분포의 피크가 높은 양의 값으로 이동함을 볼 수 있다. Figure 12에서 나타난 빈도 분포의 변화는 주로 야간에 나타난 도시열섬강도의 증가에 의한 것으로 해석된다. Figure 15는 Fig. 13에서 제시한 토지피복변화와 기상환경변화 효과를 주간과 야간으로 구분한 것이다. 주간에는 토지피복변화와 기상환경변화로 인한 10년 사이의 빈도 변화가 3% 이하로 미미하다. 반면 야간에는 Fig. 13와 유사한 빈도 변화 형태를 보인다. 야간 동안 10년 사이 빈도는 토지피복변화에 의해 1~2°C에서 가장 크게 감소하였고(−11.16%), 3~4°C와 4~5°C(각각 +8.33%)에서 가장 크게 증가하였다. 또한 기상환경변화에 의해 0~1°C의 빈도가 가장 크게 감소하였고(−3.90%), 2~3°C의 빈도가 가장 크게 증가하였다(+6.05%). 이것은 Fig. 13에서 살펴보았던 토지피복변화와 기상환경변화에 의한 열섬 강도 증가가 야간 시간대의 강도 증가로 인해 발생하였음을 의미한다.
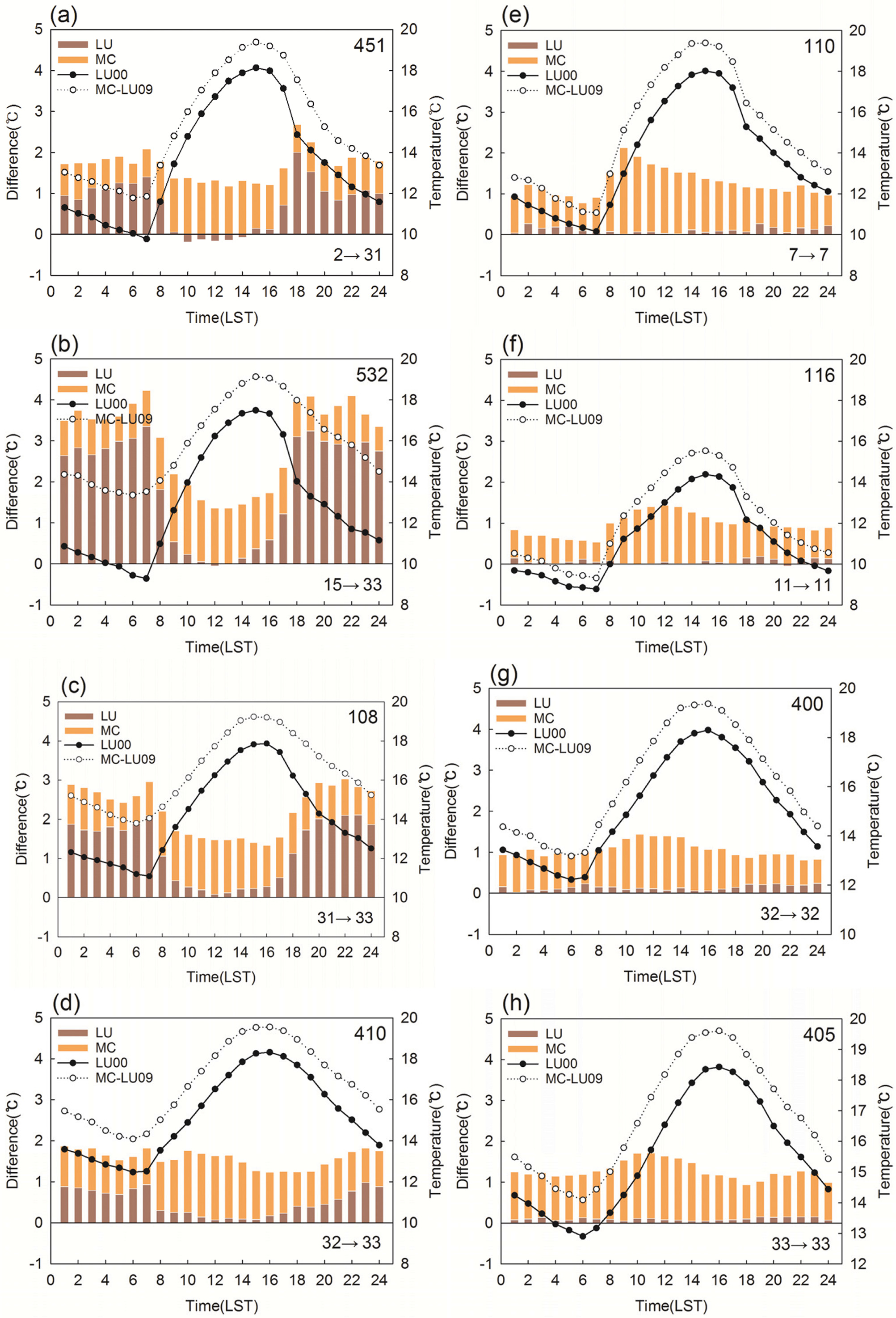
The diurnal variations of 2 m temperature over (a) Namyangju-si, (b) Uijeongbu-si, (c) Jongno-gu, (d) Dongjak-gu, (e) Gangseo-gu, (f) Gwacheon-si, (g) Gangnam-gu, and (h) Yangcheon-gu during October for LU00 (black dots and solid line) and MC-LU09 (white dots and dotted line). The brown and yellow bars denote the temperature increase by land-use change and meteorological condition change, respectively. The land-use code that was changed during 10 years is shown at the bottom right corner of each graph.
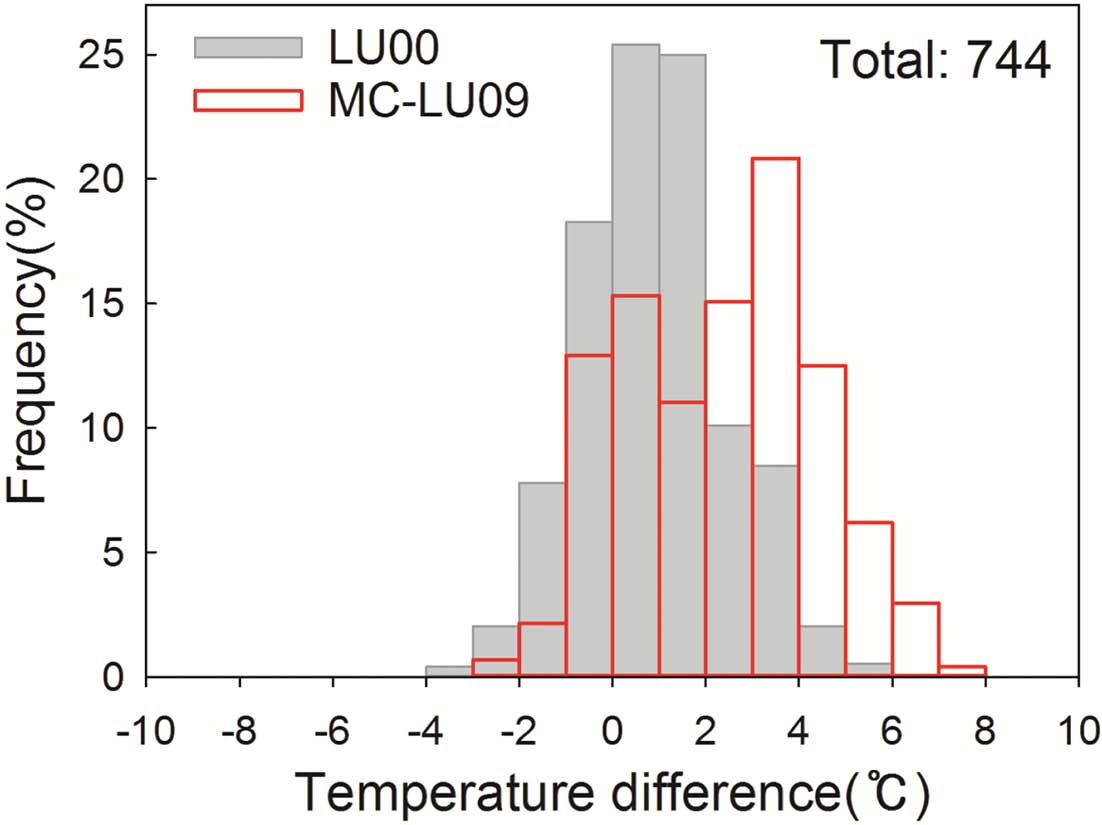
Frequency distribution of the difference between Seoul and Okcheon for hourly 2 m temperature (the urban heat island intensity) during October. The gray and red vertical bars denote LU00 and MC-LU09, respectively.
Figure 16는 수도권 중심 영역(Fig. 7의 실선)을 평균한 2000년과 2009년의 현열과 잠열플럭스의 일 변화이다. 플럭스 수송량이 많은 시간(0900~1700 LST)동안의 평균값을 살펴보면, 현열플럭스는 104.69W m−2에서 120.15W m−2로 증가하였고 잠열플럭스 또한 55.33W m−2에서 65.36W m−2로 증가하면서 10년 사이 다소 약한 변화를 보였다. 이러한 증가를 토지피복변화와 기상환경변화로 나눠서 살펴보면, 현열플럭스는 토지피복변화에 의해 4.10W m−2 증가, 기상환경변화에 의해 11.36W m−2 증가하는 결과를 보였다. 반면 잠열플럭스는 토지피복변화에 의해 −1.20W m−2 감소, 기상환경변화에 의해 11.23W m−2 증가하는 결과를 보였다.
플럭스의 일 변화 또한 각 지점별로 살펴보았다(Fig. 17). 지점은 앞서 3.2.1절에서 분석한 지점과 동일하다. 현열플럭스는 지점별 분석에서도 영역 평균(Fig. 16)과 유사하게 10년 후 플럭스 증가가 나타났다. 토지피복이 변한 지점(Figs. 17a~d)의 경우, 기상환경변화보다 토지피복변화에 의한 현열플럭스의 증가가 뚜렷하게 나타났다. 예를 들어 의정부시(532)의 시간 평균된(0900~1700 LST) 현열플럭스는 기상환경변화에 의해 4.50W m−2 증가한 반면 토지피복변화에 의해 112.48W m−2로 높게 증가하였다. 토지피복이 변하지 않은 지점(Figs. 17e~h)의 경우 현열플럭스의 미미한 증가가 나타났으며(평균 8.80W m−2) 토지피복변화와 기상환경변화의 기여도도 낮게 나타났다. 잠열플럭스의 경우, 영역 평균(Fig. 16)과는 다른 결과를 보였다. 토지피복이 변한 지점(Figs. 18a~d)의 경우, 10년 후 잠열플럭스가 감소하였다. 이러한 감소는 토지피복변화에 의해 발생하였다. 예를 들어 의정부(532)의 시간 평균된(0900~1700 LST) 잠열플럭스는 기상환경변화에 의해 28.38W m−2 증가한 반면 토지피복변화에 의해 111.13W m−2 감소하였다. 토지피복이 변하지 않은 지점(Figs. 18e~h)의 경우 현열플럭스와 유사하게 잠열플럭스에서도 미미한 증가가 나타났으며(평균 15.45W m−2) 토지피복변화와 기상환경변화의 기여도도 낮게 나타났다.
3.3 토의
수도권 지역을 대상으로 10년 사이(2000년과 2009년) 도시화로 인한 토지피복변화와 기상환경변화가 열환경에 미치는 영향을 WRF-UCM을 이용하여 분석하였다. 실험은 기본실험(2000년 토지피복지도, 2000년 10월 기상환경), 기상환경변화만을 고려한 실험(2000년 토지피복지도, 2009년 10월 기상환경), 토지피복변화와 기상환경변화를 동시에 고려한 실험(2009년 토지피복지도, 2009년 10월 기상환경)의 세 가지로 구성되었다.
본 연구는 도시의 열환경 변화를 토지피복변화 효과와 기상환경변화 효과로 나누어 정량적으로 제시하여 토지피복변화 효과만을 살펴본 기존의 수치실험 연구와 확연한 차이를 보였다. 도시화에 따른 토지피복변화와 기상환경변화가 수도권 지역의 열환경 변화에 기여하는 정도에는 차이가 있었다. 수도권 중심 영역의 2 m 기온은 토지피복변화에 의해 0.07°C에서 0.22°C, 기상환경변화에 의해 0.78°C에서 1.44°C 증가하여 토지피복변화 효과가 기상환경변화 효과에 비해 작게 나타났다. 이는 2000년에서 2009년까지의 도시 성장이 다소 작았기 때문에 영역별 평균에서 기상환경변화 효과가 주로 나타난 것으로 사료된다. 지점별로 살펴볼 경우 토지피복변화에 따른 기온 증가를 뚜렷하게 볼 수 있었다. 모든 분석 지점에서 기상환경 변화로 인한 기온 증가가 나타났으며 토지피복이 도시지역으로 변한 지점에서는 기상환경변화에 토지피복변화 효과가 더해져 야간 동안 높은 기온 증가를 보였다(최대 4.23°C). 그러나 기온의 공간분포 차이를 통해 알 수 있듯이 토지피복변화에 의해 실험 도메인 내 모든 지역에서 기온이 증가하는 것이 아니기 때문에 이에 대한 추가 연구가 필요하다. 서울과 옥천의 2 m 기온차로 정의된 도시열섬강도를 분석한 결과, 토지피복변화와 기상환경변화 모두 도시열섬강도를 강화시키는 데 기여하였으며 기상환경변화보다 토지피복변화가 도시열섬강도 강화에 더 큰 영향을 미쳤다. 지표플럭스의 경우, 기상환경변화보다 토지피복변화에 의해 명백한 변화를 보였다. 토지피복변화는 현열플럭스를 증가시키고 잠열플럭스를 감소시키는 데 기여하였으나 기상환경변화는 현열 및 잠열플럭스의 증가에 기여하였다.
본 연구는 향후 미래의 기후변화 시나리오에 대해 토지피복변화 효과와 기후변화 효과를 살펴보는 연구로 확장될 수 있다. 미래의 기후변화 시나리오는 토지피복변화 효과를 반영하지 않은 결과이기 때문에 토지피복변화 효과와 기후변화 효과를 더 정확하게 분리하여 분석할 수 있을 것으로 판단된다. 그러나 다른 관점에서 보면 토지피복변화가 수도권지역의 열환경 변화에 영향을 미치기 때문에 도시 기후를 예측할 때는 미래의 토지피복변화도 함께 고려해야 함을 시사한다.
Acknowledgments
본 연구는 기상청 국립기상과학원의 주요 사업 “차세대 도시 · 농림 융합스마트 기상서비스 개발”의 지원으로 수행되었습니다.
REFERENCES
- Bornstein, R. D., (2001), Currently used parameterisations in numerical models, Workshop on Urban Boundary layer Parameterisations, COST 715, Zurich, May 24-25 2001.
-
Chen, F., X. Yang, and W. Zhu, (2014), WRF simulations of urban heat island under hot-weather synoptic conditions: The case study of Hangzhou city, China, Atmos. Res, 138, p364-377.
[https://doi.org/10.1016/j.atmosres.2013.12.005]
-
Grossman-Clarke, S., J. A. Zehnder, T. Loridan, and C. S. B. Grimmond, (2010), Contribution of land use changes to near-surface air temperatures during recent summer extreme heat events in the Phoenix metropolitan area, J. Appl. Meteor. Climatol, 49, p1649-1664.
[https://doi.org/10.1175/2010JAMC2362.1]
- Hunt, J. C. R., D. J. Carruthers, R. E. Britter, and N. C. Daish, (2003), Dispersion from accidental releases in urban areas, Cambridge Environmental Research Consultants Ltd, Cambridge, UK.
- Jeong, J. H., and Y. K. Kim, (2009), The application of highresolution land cover and its effects on near-surface meteorological fields in two different coastal areas, J. KOSAE, 25, p432-449.
-
Jiang, X., C. Wiedinmyer, F. Chen, and Z. L. Yang, (2008), Predicted impacts of climate and land use change on surface ozone in the Houston, Texas, area, J. Geophys. Res, 113, pD20313.
[https://doi.org/10.1029/2008jd009820]
-
Kang, Y. H., Y. K. Kim, I. B. Oh, M. K. Hwang, and S. K. Song, (2010), Modeling the impacts of increased urbanization on local meteorology in the greater Seoul area, J. Korean Environ. Sci. Soc, 19, p1361-1374.
[https://doi.org/10.5322/jes.2010.19.12.1361]
- Kim, Y. H., D. Y. Choi, and D. E. Chang, (2011), Characteristics of urban meteorology in Seoul metropolitan area of Korea, Atmosphere, 21, p257-271, (in Korean with English abstract).
- Koo, H. J., Y. H. Kim, and B. C. Choi, (2007), A study on the change of the urban heat island structure in Seoul, J. Climate Res, 2, p67-78.
-
Kusaka, H., and F. Kimura, (2004), Coupling a single-layer urban canopy model with a simple atmospheric model: Impact on urban heat Island simulation for an idealized case, J. Meteor. Soc. Japan, 82, p67-80.
[https://doi.org/10.2151/jmsj.82.67]
-
Kusaka, H., H. Kondo, Y. Kikegawa, and F. Kimura, (2001), A simple single-layer urban canopy model for atmospheric models: Comparison with multi-layer and slab models, Bound.-Layer Meteor, 101, p329-358.
[https://doi.org/10.1023/A:1019207923078]
-
Lee, H. J., K. O. Lee, S. H. Lee, and H. W. Lee, (2008), Numerical study on the air cooling effect due to increased albedo in urban area, J. KOSAE, 24, p383-392.
[https://doi.org/10.5572/kosae.2008.24.4.383]
-
Lee, J. B., J. C. Kim, and Y. J. Jang, (2012), A study of urban heat island in Chuncheon using WRF model and field measurements, J. KOSAE, 28, p119-130.
[https://doi.org/10.5572/kosae.2012.28.2.119]
-
Lin, C. Y., F. Chen, J. C. Huang, W. C. Chen, Y. A. Liou, W. N. Chen, and S. C. Liu, (2008), Urban heat island effect and its impact on boundary layer development and land-sea circulation over northern Taiwan, Atmos. Environ, 42, p5635-5649.
[https://doi.org/10.1016/j.atmosenv.2008.03.015]
- Meng, W. G., Y. X. Zhang, J. N. Li, W. S. Lin, G. F. Dai, and H. R. Li, (2011), Application of WRF/UCM in the simulation of a heat wave event and urban heat island around Guangzhou, J. Trop. Meteorol, 17, p257-267.
- Mitchell, K., (2000), The community Noah land-surface model (LSM): users guide, Technical Report, National Centers for Environmental Prediction (NCEP), USA.
- Moon, Y. S., and Y. S. Koo, (2006), A study on examples applicable to numerical land cover map data for atmospheric environment fields in the metropolitan area of Seoul - Real time calculation of biogenic CO2 flux and VOC emission due to a geographical distribution of vegetable and analysis on sensitivity of air temperature and wind field within MM5, J. KOSAE, 22, p661-678.
- National Center for Atmospheric Research (NCAR), (2005), PSU/NCAR mesoscale modeling system tutorial class notes and user’s guide: MM5 modeling system version 3..
-
Pathirana, A., H. B. Denekew, W Veerbeek, C. Zevenbergen, and A. T. Banda, (2014), Impact of urban growth-driven landuse change on microclimate and extreme precipitation - A sensitivity study, Atmos. Res, 138, p59-72.
[https://doi.org/10.1016/j.atmosres.2013.10.005]
-
Wang, X., Z. Wu, and G. Liang, (2009a), WRF/CHEM modeling of impacts of weather conditions modified by urban expansion on secondary organic aerosol formation over the Pearl River Delta, Particuology, 7, p384-391.
[https://doi.org/10.1016/j.partic.2009.04.007]
-
Wang, X., F. Chen, Z. Wu, M. Zhang, M. Tewari, A. Guenther, and C. Wiedinmyer, (2009b), Impacts of weather conditions modified by urban expansion on surface ozone: Comparison between the Pearl River Delta and Yangtze River Delta regions, Adv. Atmos. Sci, 26, p962-972.
[https://doi.org/10.1007/s00376-009-8001-2]